Volume 12 - Year 2025 - Pages 156-161
DOI: 10.11159/jffhmt.2025.017
Bioenergy from Biomass as an Ecuador-Peru Border Circular Economy Strategy
Hugo Romero-Bonilla1, Cristhian Vega-Quezada2, Joseph Cruel3, Jose Mamani-Quispe4, María Farias-Gonsalez1, Cristopher Choez-Tobo1
1Faculty of Chemical and Health Sciences. Technical University of Machala Machala-Ecuador.
E-mail: hromero@utmachala.edu.ec; mfarias@utmachala.edu.ec; cchoez1@utmachala.edu.ec
ORCID: https://orcid.org/0000-0002-7846-0512; https://orcid.org/0009-0008-7666-0941; https://orcid.org/0009-0004-5761-6161
2Faculty of Business Sciences. Technical University of Machala. Machala-Ecuador.
E-mail: cvega@utmachala.edu.ec
ORCID: https://orcid.org/0000-0002-7525-2486
3Faculty of Engineering. 'Luis Vargas Torres' Technical University. Esmeraldas, Ecuador.
E-mail: josephcruel1983@gmail.com
ORCID: https://orcid.org/0000-0002-3949-0049
4Faculty of Chemical Engineering. Universidad del Altiplano. Puno, Perú.
E-mail: jomamani@una.edu.pe
ORCID: https://orcid.org/0000-0001-7803-7936
Abstract - This study reviews recent advances in the use of biodegradable waste for bioenergy purposes and employs the concept of the circular economy to assess regional development strategies grounded in the bioeconomy within the border region of Ecuador and Peru. Stratified sampling by clusters, the estimation of CO2 equivalent emissions, gas chromatography as an instrumental analytical method for methane quantification, and the electrical conversion of biogas are the methodological tools applied in this work. Among the estimated results, it can be mentioned that, due to the emissions of unused MSW, the opportunity cost for non-mitigation of its CO2 emissions is estimated at 0.34 and 2.58 million dollars at the local and regional level respectively. From 1.5 ton with recirculation of the biodegradable solid waste leachate, a maximum methane bioconversion of 93.89% purity in the biogas was achieved in phase 2 and 70.73% in Phase 1 after 60 days and 50 days in Phase 2. With this bioenergy potential, significant monetary benefits can be achieved, as well as an approximate NPV between 453 and 3,426 million dollars by implementing this initiative at the local and regional level respectively.
Keywords: Circular economy, biogas, CO2 emissions, bioenergy, gas chromatography, biowaste.
© Copyright 2025 Authors - This is an Open Access article published under the Creative Commons Attribution License terms Creative Commons Attribution License terms. Unrestricted use, distribution, and reproduction in any medium are permitted, provided the original work is properly cited.
Date Received: 2023-11-10
Date Revised: 2023-12-04
Date Accepted: 2024-04-09
Date Published: 2025-05-08
1. Introduction
The management of municipal solid waste (MSW) and agricultural solid waste is a global concern, particularly in regions experiencing significant population growth [1]. This poses a negative impact on human health and the environment [2]. In Latin America and the Caribbean (LAC), MSW coverage stands at 89%, while the adequate final disposal service (in sanitary landfills) for MSW is approximately 55%. This means that in LAC, a significant proportion of waste (45%) is not adequately disposed of and/or treated. With the regional per capita generation of Domestic Solid Waste (DSW) relative to MSW being 0.6 and 0.9 (kg/capita/day), respectively, it becomes an environmental problem due to greenhouse gas emissions from the substantial amount of biodegradable waste [3]. In Ecuador, around 0.84 kg/day of urban solid waste per inhabitant was produced in 2019, with Guayas being the highest-demand province at 1.03 kg/day, followed by Los Rios at 0.90 kg/day, among other provinces like Pichincha, generating 0.88 kg/day, and El Oro and Santo Domingo de los Tsáchilas provinces at 0.81 and 0.80 kg/day, respectively [4].
Currently, considering biodegradable waste as a byproduct within the framework of the circular economy is an innovative approach of scientific interest, reflected in the growing research related to the use, technologies, and new byproducts of urban and agricultural biodegradable waste treatment [5]. From the extensive current literature, the use of biological waste in co-gasification processes stands out [6].
Biohydrogen, as a bioenergy source, is of great relevance, as it is obtained from biomass in solid waste, which is often biological (dark fermentation) or thermochemical. Sanitary landfills using solid waste for biogas generation are used for electricity production. According to reports from 2020, Mexico could generate around 1629 to 2248 tons/year of Methane (CH4) and produce approximately 652 to 912 MW of energy [7].
In the case of LAC, current studies related to the treatment of biodegradable waste include works such as that by [8], integrating a set of systemic initiatives related to the bioenergy potential in Ecuador concerning urban and agricultural waste utilization (illustration 1). This work comprises three additional sections. The first describes the methodological tools used in the case study, such as stratified cluster sampling, experimental design under operational conditions, gas chromatography, biogas electrical conversion, and cost-benefit analysis.
Method
Sampling, Equivalent CO2 Emissions, and Biogas Electrical Conversion
For the identification and characterization of the availability of biodegradable waste, a stratified cluster sampling method was employed, utilizing a sample of 940 bags of municipal solid waste (MSW). The strata corresponded to five zones based on the city's census distribution. The number of MSW bags collected was proportional to the number of households in each zone, with systematic sampling every 5 households within each zone.
The quantification of bovine manure availability (CM) was estimated based on livestock inventory, applying the methodology from [9] using the following formula:

Where the animal population signifies the count of animal units per 1000 kg of weight [10]; VS refers to the volume excreted by the type of animal in a year expressed in kilograms; MSW denotes the type of manure management system according to agricultural and livestock exploitation during the production process, expressed as a percentage. The equivalent CO2 emissions that are avoided through the management of bovine manure are quantified using the methodology from [9], measuring CH4 and NOX emissions following the work of [8], expressed in teragrams (Tg) of CO2-equivalent.
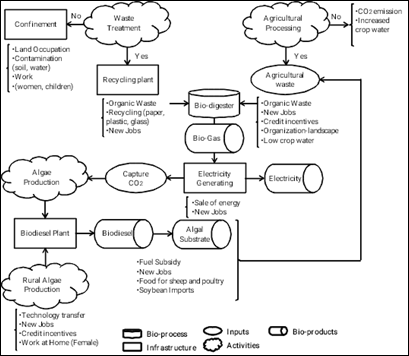
Estimations of potential CO2 emissions resulting from biogas combustion in electricity generation are performed based on the research conducted in [11]. This study calculates the energy potential of cattle manure biogas (E_biogas) expressed in Billions of BTU per year, the electrical potential from biogas (_biogas) in kilowatt-hours (kWh), the total carbon dioxide emissions from the combustion of one cubic meter of biogas (kg (CO2 total)), and the amount of kg of CO2 emitted per kWh of electricity generated during its combustion.
Experimental System
In the initial phase, three 20-liter capacity reactors (drums) were inoculated with different mixtures of biodegradable waste, all of which were supplemented with cattle manure and 2 liters of tap water, totaling 4 kg of wet biomass (Table 1). The biogas primarily consisted of CO2 and CH4, greenhouse gases of which we needed to determine the concentration in the generated biogas to meet the research objectives. This biogas was stored in a polypropylene bag for subsequent gas chromatography analysis. The total experimental duration of the first phase was 12 days.
For the second phase of the research, a 1.5-ton capacity digester was utilized, following the technical and operational characteristics required for two-phase anaerobic digestion as per the study by [12]. The biogas production volume and CH4/m3 content were regularly measured. The retention time for this phase was 50 days, employing the biomass composition shown in Table 2.
Table 1. Experimental Conditions for the first phase of research
Reactor 1A |
Reactor 1B |
Reactor 1C |
0.5 kg potato peel + |
0.5 kg parsley + |
0.5 kg bean husks + |
0.5 kg carrot |
0.5 kg white onion |
0.5 kg pea husks |
1.0 kg cow manure |
1.0 kg cow manure |
1.0 kg cow manure |
2.0 L water |
2.0 L water |
2.0 L water |
Table 2. Experimental conditions for the second phase of the research
Solid phase 1 |
Liquid phase 2 |
167.0 kg pea husks + |
120 L Landfill leachate |
133.0 kg vean husks |
3.0 kg biofilms - inert material |
100.0 kg cow manure |
|
600.0 L water |
|
Gas Chromatographic Analysis of CO2 and CH4
Gas concentrations produced during each phase of the reactor experiments were measured daily using the methodology described by [13], following the optimization of chromatographic parameters.
Cost-Benefit Analysis
To evaluate the comprehensive environmental, social, and economic advantages, these are quantified in monetary terms through private and public cost-benefit analyses. This assessment considers the criteria of Net Present Value (NPV) and the Benefit-Cost Ratio (BCR). It employs an annual discount rate of 8.64% and extends the time horizon up to the year 2028, covering a period of 14 years for monetary flows, following the framework proposed by [13].
Regarding environmental benefits, the equivalent CO2 emissions due to manure management [9] and the water demand for co-digestion of MSW + CM in biogas production [14] are also estimated.
All estimations are made at the local level and extrapolated to the regional level based on the number of inhabitants and the available livestock inventory from official information sources [15], considering the limitations and implications of this assumption.
3. RESULTS
Assessment of the anaerobic digestion process in the first stage
The average values of the percentages of CO2 and CH4 obtained during the first phase of anaerobic digestion of the 3 types of biomass mixtures studied in batch anaerobic reactors after 12 days of experimentation are shown in Table 3. It can be observed that Reactor 1C produced the highest percentage of methane.
Tabla 3. CO2 and CH4 obtained during anaerobic digestion by types of mixture
|
Reactor1A |
|
Reactor1B |
|
Reactor1C |
|
Days |
CO2 (%) |
CH4 (%) |
CO2 (%) |
CH4 (%) |
CO2 (%) |
CH4 (%) |
12 |
96.53 |
3.47 |
98.89 |
1.11 |
86.47 |
13.33 |
Based on the higher methane yield obtained in reactor 1C, this type of biomass was selected for the second phase.
Evaluation of the Anaerobic Digestion Process in the Second Stage
Table 4 displays the results of CO2 and CH4 conversion from biodegradable waste of beans and peas co-digested with bovine manure in a two-phase reactor: the first solid phase with a capacity of 1.5 tons with leachate recirculation and the second liquid phase with four columns containing support material and inoculum with methanogenic bacteria that receive effluents rich in volatile fatty acids (VFA) with excess discharge to the solid phase reactor [12]. It was observed that after 60 days in Phase 1 and 50 days of experimentation in Phase 2, a maximum methane conversion of 93.89% purity in the biogas in Phase 2 and 70.73% in Phase 1 was achieved.
Tabla 4. CO2 and CH4 obtained during anaerobic digestion in a two-phase digester
Days |
|
CO2 (%) |
CH4 (%) |
60 - 50 |
Solid phase 1 |
29.24 % |
70.73 % |
Liquid phase 2 |
5.41 % |
93.89 % |
Economic Benefits
Based on the information presented in the previous sections, economic benefits for the proposed systemic framework are estimated at both the local and regional levels. Table 5 summarizes the set of estimated indicators, starting with the economic indicators related to utilizing MSW + CM in biogas production for energy purposes. The primary result highlights that electricity production from biogas as a standalone purpose is not financially attractive. However, when incorporating electricity production as a component of a holistic approach in line with the circular economy concept, the byproducts of anaerobic digestion in biogas production generate significant monetary benefits. The estimated NPV is approximately between 453 and 3426 million dollars upon implementing this initiative at the local and regional levels, respectively.
Among the environmental indicators in Table 5, equivalent CO2 emissions generated by MSW, manure management, and biogas combustion for electricity generation are presented. The total estimated emissions amount to 1.70 million tons at the local level and 12.99 million tons at the regional level. Additionally, potential economic benefits for mitigating these emissions through initiatives like the one proposed in this study are provided. The cost of opportunity for not mitigating CO2 emissions is estimated at 0.34 and 2.58 million dollars at the local and regional levels, respectively.
Table 5. Economic Indicators in the Use of MSW + CM Considering Biogas Production for Energy Purposes, Under the Circular Economy Approach.
Description |
Area |
|
Local (Millions of dollars) |
Regional (Millions of dollars) |
|
Economic indicators |
|
|
(+) Income from electricity sales |
6.58 |
49.76 |
(-) Biodigester + electric generators |
18.62 |
142.73 |
(-) Maintenance and operation |
1.09 |
9.63 |
(-) Labor cost for the biodigester and electricity generation |
372.26 |
2820.35 |
(+) Liquid fertilizer |
767.78 |
5816.97 |
(+) Solid fertilizer |
70.23 |
532.09 |
(=) NPV |
452.63 |
3426.12 |
Opportunity cost |
|
|
(+/-) MSW CO2 Emissions |
0.27 |
2.05 |
(+/-) CO2 Emissions – Manure Management |
0.02 |
0.19 |
(+/-) CO2 Emissions – Biogas Combustion |
0.05 |
0.34 |
(=) NPV |
0.34 |
2.58 |
4. Discussion
From the experimental phase, it is important to note that in stage 1 the reactor with the mixture of bean husks + pea husks + cow manure presented the highest methane production, while in stage 2 with the 2-phase prototype the Biogas production reached 11.9 m3, which is significantly lower compared to the 96.6 m3 reported by [12] in their study.
This difference generates concern, despite achieving an approximate 71% reduction in COD compared to its original value. Therefore, for future research it is necessary to review and validate the hermetic conditions of the biodigester and its connections up to the biogas sampling point. However, regarding the average percentage of CH4/m3 of biogas, we were able to achieve the performance of 70% or more reported by [12].
As part of future research, it is important to consider the nitrogen, phosphorus and potassium content in the digested biomass of the prototype in phase 1 and the leachate resulting from phase 2 since their bioeconomic potential as biofertilizers is important.
5. Conclusions
This work allows us to infer that designing bioenergy initiatives as isolated activities, without identifying synergies and interactions with byproducts, is not economically viable compared to implementing sets of initiatives within the framework of the circular economy.
It was determined that, due to the emissions of unused MSW, the opportunity cost of not mitigating its CO2 emissions is estimated at 0.34 and 2.58 million dollars at the local and regional level, respectively. With 1.5 tons of biodegradable solid waste leachate recirculation, a maximum methane bioconversion of 93.89% purity into biogas was achieved in phase 2 and 70.73% in phase 1 after 60 days in phase 1 and 50 days in phase 2. This bioenergy potential can result in significant monetary benefits, along with an estimated NPV of approximately 453 and 3,426 million dollars when implementing this initiative at the local and regional levels, respectively. Becoming an initiative with high development potential from the edges for countries like Peru and Ecuador.
References
[1] M. Sharholy, K. Ahmad, G. Mahmood y R. Trivedi, «Municipal solid waste management in Indian cities - A review,» Waste Management, vol. 28, nº 2, pp. 459-467, 2008. View Article
[2] S. Khan, R. Anjum, S. T. Raza, N. A. Bazai y M. Ihtisham, «Technologies for municipal solid waste management: Current status, challenges, and future perspectives,» Chemosphere, vol. 288, 2022. View Article
[3] J. Grau, H. Terraza, D. Rodríguez, A. Rihm y G. Sturzenegger, «Solid Waste Management in Latin America and the Caribbean,» 2015. View Article
[4] J. Aguirre López y J. C. Ortega Castro, «Costos Operativos de los sistemas de recolección de residuos sólidos en los cantones de Ecuador,» FIPCAEC, vol. 7, nº 1, pp. 412-429, 2022.
[5] N. Howaniec y A. Smoliński, «Biowaste utilization in the process of co-gasification with bituminous coal and lignite,» Energy, vol. 118, pp. 18-23, 2017. View Article
[6] S. Paniagua, J. Clavijo, R. Orellana y D. Sempértegui, «EVALUACIÓN DE LA GENERACIÓN DE ENERGIA A PARTIR DE LA GASIFICACIÓN DE RESIDUOS SÓLIDOS URBANOS EN COCHABAMBA-BOLIVIA,» INVESTIGACIÓN & DESARROLLO, vol. 22, nº 1: 25-36, 2022. View Article
[7] E. Sosa, «Alternativas bioenergéticas de los residuos sólidos urbanos: panorama en México,» Letras Verdes. Revista Latinoamericana De Estudios Socioambientales, 2022.
[8] C. Vega, M. Blanco y H. Romero, «Synergies between agriculture and bioenergy in Latin American countries: A circular economy strategy for bioenergy production in Ecuador,» de New Biotechnology, vol. 39, 2017, pp. 81-89. View Article
[9] «INVENTORY OF U.S. GREENHOUSE GAS EMISSIONS AND SINKS: 1990-2020,» EPA. United States Environmental Protection Agency, 13 Abril 2023.
[10] R. Kellogg, C. Lander, D. Moffitt y N. Gollehon, «Manure Nutrients Relative to the Capacity of Cropland and Pastureland to Assimilate Nutrients: Spatial and Temporal Trends for the United States,» USDA. United States Department of Agriculture, 2000. View Article
[11] A. D. Cuéllar y M. E. Webber , «Cow power: the energy and emissions benefits of converting manure to biogas,» Environmental Research Letters, vol. 3, nº 3. View Article
[12] M. Macias, Z. Samani, A. Hanson, G. Smith, P. Funk, H. Yu y J. Longworth, «Anaerobic digestion of municipal solid waste and agricultural waste and the effect of co-digestion with dairy cow manure,» Bioresource Technology, vol. 99, pp. 8288-8293, 2008. View Article
[13] C. Vega, M. Blanco y H. Romero, «Production of Bioenergy in the Framework of Circular Economy: A Sustainable Circular Systemin Ecuador.,» de Advances in Biofeedstocks and Biofuels, vol. 1, L. Kumar y G. Chaudhary, Edits., Scrivener Publishing/Wiley, 2017, pp. 1-32. View Article
[14] C. Castillo y C. Del Valle Jurado, «Evaluación del reaprovechamiento de los residuos sólidos orgánicos generados por los servicios de un catering,» Revista Del Instituto De investigación De La Facultad De Minas, Metalurgia Y Ciencias geográficas,, vol. 26, nº 51, 2023. View Article
[15] INEC, «Viewer Ecuador Agricultural Statistics ESPAC,» 2022. [En línea]. Available: View Article