Volume 12 - Year 2025 - Pages 105-109
DOI: 10.11159/jffhmt.2025.011
Impact of BiBO3, NaF and BiF3 Substitution on the Thermoelectric Properties of Bi2Ca2Co2Oy Ceramics
Iamze Kvartskhava1, Nikoloz Margiani1, Vakhtang Zhghamadze1, Giorgi Kakhniashvili1, Natia Margiani1, and Irina Khomeriki2
1Georgian Technical University, Institute of Cybernetics, Z. Anjaparidze str., 1st
Ln., N6, 0186, Tbilisi, Georgia,
iakvartskhava@gmail.com, n.margiani@gtu.ge, vakhtang66@gmail.com, g.mumladze@gtu.ge, georgekakhniashvili13081955@gmail.com, natiamargiani@gmail.com
2Georgian Technical University, Faculty of Informatics and Control Systems, 77
Kostava str., 0160, Tbilisi, Georgia,
i.khomeriki@gtu.ge
Abstract - In this work, we studied the impact of (i) partial substitution of bismuth oxide (Bi2O3) by bismuth borate (BiBO3), (ii) dual-substitution of Bi2O3 by BiBO3 and sodium fluoride (NaF), and (iii) dual-substitution of Bi2O3 by BiBO3 and bismuth fluoride (BiF3) on the thermoelectric characteristics of Bi2Ca2Co2Oy layered cobaltite. Thermoelectric cobaltites were synthesized using the sol-gel method. The phase composition of prepared materials was examined by the X-ray diffraction (XRD) analysis. The values of power factor (PF) and figure of merit (ZT) were calculated through measurements of electrical resistivity (ρ), Seebeck coefficient (S), and thermal conductivity (k). The XRD analysis confirms that all the samples consist of a nearly pure Bi2Ca2Co2Oy phase. When compared to the reference (pristine) sample, the dual NaF/BiBO3 substitution leads to a sharp decrease in ρ due to the partial substitution of NaF for Bi2O3, which increases the concentration of charge carriers (holes). At the same time, partial substitution of BiF3 for Bi2O3 led to a decrease in hole concentration and, hence, an increase in ρ. Seebeck coefficients were positive for all the samples, indicating p-type conductivity. The maximum PF and ZT values achieved in the NaF/BiBO3 co-substituted composition are 18% and 13% higher, respectively, than the reference Bi2Ca2Co2Oy.
Keywords: Thermoelectricity, Substitution, Sol-gel technique, Power factor, Figure of merit.
© Copyright 2025 Authors - This is an Open Access article published under the Creative Commons Attribution License terms Creative Commons Attribution License terms. Unrestricted use, distribution, and reproduction in any medium are permitted, provided the original work is properly cited.
Date Received: 2025-08-22
Date Revised: 2025-02-17
Date Accepted: 2025-03-02
Date Published: 2025-03-25
1. Introduction
Due to the increasing global energy demand and climate change issues, the importance of environmentally friendly renewable energy technologies is growing. Many studies in this field focus on thermoelectric materials that can directly convert waste heat into electric power. The discovery of thermoelectric properties in complex cobalt oxides, NaCo2O4, Ca3Co4O9 and Bi2M2Co2O (M = Ca, Sr or Ba), opened the way to the systematic exploration and development of polycrystalline cobaltites for potential applications [1]-[4]. Metal oxides offer advantages over conventional non-oxide thermoelectric materials (intermetallic compounds and alloys) due to their environmentally friendliness, ease of processing, high thermal and chemical stability, abundance, and low cost of raw materials. The thermoelectric conversion efficiency of materials is characterized by the dimensionless figure-of-merit ZT = S2T/ρk, where S represents the Seebeck coefficient, T is the absolute temperature, ρ denotes electrical resistivity, and k stands for total thermal conductivity [5]. The power factor PF = S2/ρ is an electrical component of the ZT formula that assesses output electrical power. However, a significant drawback of thermoelectric cobaltites is their low heat-to-electricity conversion efficiency compared to conventional materials [6]. Therefore, the challenge lies in improving electronic transport properties, such as electrical conductivity and the Seebeck coefficient, while also minimizing the phonon contribution to thermal transport by carefully selecting scattering centers [7]. Various strategies can improve the efficiency of thermoelectric oxides, such as texturing, soft-chemistry techniques, and the incorporation of substituents and additives into these materials [8]-[12]. The sol-gel synthesis provides a promising method to enhance the conversion efficiency of thermoelectric materials by achieving exceptionally high chemical homogeneity. One of the main advantages of gel chemistry is its use of well-mixed solutions of molecular precursors, which ensures consistent homogeneity throughout the entire process, from the initial solution to the final product. Additionally, the high versatility of the sol-gel technique allows for easy modification of both the type and concentration of the substituents and additives [13]. The p-type Bi2Sr2Co2Oy and Bi2Ca2Co2Oy cobaltites are promising materials for thermoelectric applications [14]. Sol-gel-derived Bi2Sr2Co2Oy and Bi2Ca2Co2Oy showed better characteristics than those made by the conventional solid-state reaction technique [15]-[18]. We recently reported an improvement in the ZT of the bismuth borate (BiBO3)-incorporated Bi2Sr2Co2Oy, which was prepared by the sol-gel method [12]. It was shown that the ZT value of BiBO3-added Bi2Sr2Co2Oy increases by 39 % at 573 K compared to the reference specimen. In this work, we studied the impact of (i) partial substitution of bismuth oxide (Bi2O3) by BiBO3, (ii) dual-substitution of Bi2O3 by BiBO3 and sodium fluoride (NaF), and (iii) dual-substitution of Bi2O3 by BiBO3 and bismuth fluoride (BiF3) on the thermoelectric characteristics of Bi2Ca2Co2Oy layered cobaltite.
2. Materials and Methods
The Reference, BiBO3–substituted as well as BiBO3/NaF and BiBO3/BiF3 dual substituted samples with the nominal compositions: Bi2Ca2Co2Oy (reference), Bi1.9925Ca2Co2(BiBO3)0.0075Oy,
Bi1.8925Ca2Co2(NaF)0.10(BiBO3)0.0075Oy, and
Bi1.8925Ca2Co2(BiF3)0.10(BiBO3)0.0075Oy were prepared by the sol-gel method using appropriate amounts of Bi(NO3)3‧5H2O, (≥98 %, Thermo Scientific), CaCO3 (≥99 %, Sigma-Aldrich), Co(NO3)2‧6H2O (≥99 %, Thermo Scientific), BiBO3 (≥99 %, RESEARCH-LAB), NaF (≥99.9 %, Sigma-Aldrich), and BiF3 (99 %, Thermo Scientific) powders as starting materials with citric acid (C6H8O7) and ethylene glycol (С2H6O2) as chelating agents. Afterwards, the 4 batches were moved to a magnetic hot plate stirrer (SMHS-3, Nabitex Scientific GmbH). The stirring rate was set from 400 to 500 rpm, and the temperature was maintained at 363 K for approximately 50 minutes. The substance levels were carefully monitored until the mixture reached a gel-like consistency with a sharp pink colour. The gels were then transferred to alumina crucibles and dried in an oven at a temperature range of 353 to 443 K for 4 h. Once the gel masses turned brown and stopped expanding in the crucibles, thermal processing continued by heating the materials to 773 K to burn off any excess organic compounds . The resulting materials were ground manually in an agate mortar to break the agglomerates, followed by calcination at temperatures ranging from 1033 to 1068 K for 25 hours in a furnace, with intermediate grinding. Finally, the calcined powders were pressed into pellets at 220 MPa and sintered at 1098 K for 17 h. The density of pellets was determined by Archimedes' method, taking 6.35 g/cm3 as the theoretical value [19]. The phase composition of the prepared materials was analyzed using X-ray diffraction (XRD, Dron–3M diffractometer, Cu Kα-radiation). The temperature dependence of the resistivity ρ(T) and Seebeck coefficient S(T) was measured simultaneously from room temperature to 973 K with a laboratory-made setup equipped with a KEITHLEY DMM6500 multimeter. Electrical transport measurements were performed on bar-shaped samples with dimensions of ~13×7×2.5 mm3. Thermal conductivity was determined from 300 to 573 K using the "Hot Disk TPS 500 thermal constants analyzer". Finally, values of PF and ZT were calculated to assess the thermoelectric performance of the synthesized cobaltite materials.
3. Results and Discussion
Figure 1 displays the XRD patterns of the prepared compositions, which closely match previously reported results for the Bi2Ca2Co2Oy system [8]-[9]. Only trace amounts of secondary phases were detected.
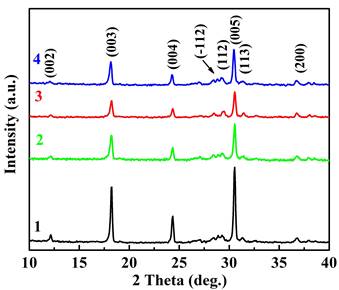
2 – Bi1.9925Ca2Co2(BiBO3)0.0075Oy,
3 – Bi1.8925Ca2Co2(NaF)0.10(BiBO3)0.0075Oy,
4 – Bi1.8925Ca2Co2(BiF3 )0.10(BiBO3)0.0075Oy.
The densities of the prepared materials are similar and correspond to approximately 96-98 % of the theoretical value. Figure 2 illustrates the temperature dependence of the resistivity, Seebeck coefficient and power factor. Both the single BiBO3-substituted and double NaF/BiBO3-substituted materials exhibit a decrease in resistivity, which is advantageous for enhancing thermoelectric performance. The lowest resistivity value at 973 K, equal to 29.5 mΩ‧cm, was found for the NaF/BiBO3 double-substituted sample, which is 1.3-fold smaller than for reference Bi2Sr2Co2O. This result is due to the partial substitution of NaF for Bi2O3, which increases the concentration of charge carriers (holes). At the same time, partial substitution of BiF3 for Bi2O3 led to a decrease in hole concentration and, hence, an increase in ρ. Seebeck coefficients were positive for all the samples, indicating p-type conductivity. The value of S for the NaF/BiBO3 co-substituted composition at 973 K is 4–6 % lower than that of the reference, BiBO3-substituted, and BiF3/BiBO3 co-substituted samples. Positive values of the Seebeck coefficient, indicating p-type conductivity in all samples, increase as the temperature rises above 480 K (Figure 2b). Combining the values of the S and ρ, the PF was calculated and plotted in Figure 2c. The maximum PF value attained in the NaF/BiBO3 double-substituted composition, 0.16 mW/(m‧K2), is 18% higher than the reference Bi2Ca2Co2Oy. Consequently, the PF of the NaF/BiBO3 double-substituted sample exceeds the values reported in the literature for both reference and doped Bi2Ca2Co2Oy compositions. These compositions were prepared via various methods, including the sol-gel process [11],[17], conventional solid-state sintering [17],[20] and a combination of high-energy ball milling followed by hot pressing sintering [9].
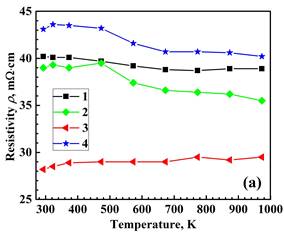
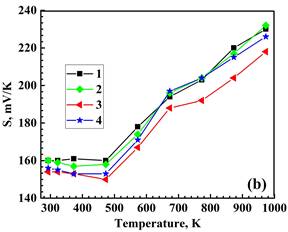
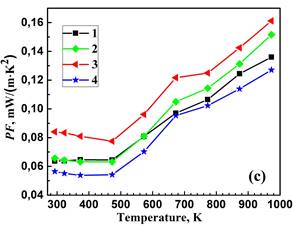
2 – Bi1.9925Ca2Co2(BiBO3)0.0075Oy,
3 – Bi1.8925Ca2Co2(NaF)0.10(BiBO3)0.0075Oy,
4 – Bi1.8925Ca2Co2(BiF3 )0.10(BiBO3)0.0075Oy.
Figure 3 displays the measured total thermal conductivity, lattice (phonon) klatt and electronic kel components of total thermal conductivity, and the calculated figure of merit for the prepared materials from 293 to 573 K. The electronic thermal conductivity, kel was determined through the Wiedemann–Franz law as kel = LT/ρ, where L=2.44 × 10-8 V2‧K-2 is the Lorenz number, T is the absolute temperature, and ρ is the electrical resistivity [9]. Then, the phonon thermal conductivity was separated from the total thermal conductivity as kph = k−kel. Double BiBO3/NaF substitution increases the k value of the Bi2Ca2Co2Oy system while the single BiBO3 and double BiBO3/BiF3 substituents cause the decrease of k. In all samples, kel is significantly lower than total thermal conductivity, with kph dominating in the k. Double BiBO3/NaF substituted sample possesses a higher ZT value than the reference sample due to an increased electrical conductivity. The ZT for double BiBO3/NaF substituted samples reaches 0.036 at 573 K, which is 13 % higher than that of the reference Bi2Ca2Co2Oy. This value of ZT is higher than previously reported in [18] for the solid-state and sol-gel processed Bi2Ca2Co1.7Oy and lies between sol-gel and polymer solution methods.
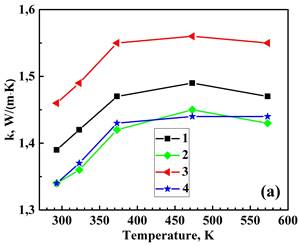
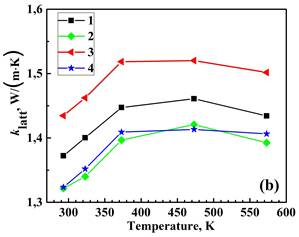
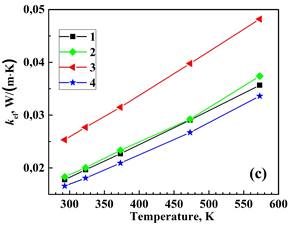
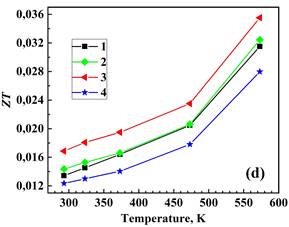
2 – Bi1.9925Ca2Co2(BiBO3)0.0075Oy,
3 – Bi1.8925Ca2Co2(NaF)0.10(BiBO3)0.0075Oy,
4– Bi1.8925Ca2Co2(BiF3 )0.10(BiBO3)0.0075Oy.
4. Conclusion
We investigated the sol-gel derived Bi₂Sr₂Co₂Oy samples that were either partially substituted with BiBO₃ or partially co-substituted with BiBO₃/NaF and BiBO₃/BiF₃. The maximum PF and ZT values of the NaF/BiBO₃ co-substituted composition were 18 % and 13 % higher, respectively, compared to the reference Bi₂Ca₂Co₂Oy. Identifying the optimal amounts of substituents could further enhance the thermoelectric performance of Bi₂Ca₂Co₂Oy cobaltite.
Acknowledgements
This work was supported by the International Science and Technology Center (ISTC) [Project # GE-2776/Enhancing the thermoelectric conversion performance of cobalt-based oxide materials through doping and microstructure modulation].
References
[1] I. Terasaki, Y. Sasago, K. Uchinokura. Large thermoelectric power in NaCo2O4 single crystals. Physical Review B. 1997, 56, R12685–R12687. DOI: https://doi.org/10.1103/Phys. View Article
[2] R. Funahashi, I. Matsubara, H. Ikuta, T. Takeuchi, U. Mizutani, S. Sodeoka. An oxide single crystal with high thermoelectric performance in air. Japanese Journal of Applied Physics. 2000, 39, L1127–L1129. https://dx.doi.org/10.1143/JJAP.39.L1127. View Article
[3] C. Masset, C. Michel, A. Maignan, M. Hervieu, O. Toulemonde, F. Studer, B. Raveau, J. Hejtmanek. Misfit-layered cobaltite with an anisotropic giant magnetoresistance: Ca3Co4O9. Physical Review B. 2000, 62, 166–175. DOI:https://doi.org/10.1103/PhysRevB.62.166. View Article
[4] R. Funahashi, I. Matsubara, S. Sodeoka. Thermoelectric properties of Bi2Sr2Co2Ox polycrystalline materials. Applied Physics Letters. 2000, 76, 2385–2387. https://doi.org/10.1063/1.126354. View Article
[5] He, J.; Liu, Y.; Funahashi, R. Oxide thermoelectrics: The challenges, progress, and outlook. Journal of Materials Research. 2011, 26, 1762-1772. https://doi.org/10.1557/jmr.2011.108. View Article
[6] K. Fujimoto, M. Gibu, Y. Yamaguchi, A. Aimi, K. Nishio, O. Rabin, I. Takeuchi. Thermoelectric properties of bismuth-substituted calcium manganite Ca1−xBixMnO3−δ prepared via the electrostatic spray deposition method. Journal of the Ceramic Society of Japan. 2017, 125, 308-312. DOI: https://doi.org/10.2109/jcersj2.16277. View Article
[7] M. Ohtaki, Recent aspects of oxide thermoelectric materials for power generation from mid-to-high temperature heat source. Journal of the Ceramic Society of Japan. 2011, 119, 770-775. https://doi.org/10.2109/jcersj2.119.770. View Article
[8] Can Özçelik, Tolga Depci, Mehmet Gürsul, Andres Sotelo. Drastic microstructural modification of Bi2Ca2Co2Oy ceramics by Na doping and laser texturing. 2022, Boletin de la Sociedad Espanola de Ceramica y Vidrio. 61, 634-640, https://doi.org/10.1016/j.bsecv.2021.06.003. View Article
[9] Song-Tao Dong, Miao-Cheng Yu, Zhuang Fu, Yang-Yang Lv, Shu-Hua Yao, Y.B. Chen, High thermoelectric performance of NaF-doped Bi2Ca2Co2Oy ceramic samples, 2022, Journal of Materials Research and Technology, 17, 1598-1604, https://doi.org/10.1016/j.jmrt.2022.01.102. View Article
[10] M.A. Madre, Sh. Rasekh, K. Touati, C. Salvador, M. Depriester, M.A. Torres, P. Bosque, J.C. Diez, A. Sotelo, From nanosized precursors to high performance ceramics: The case of Bi2Ca2Co1.7Ox, Materials Letters. 2017, 191, 14-16, https://doi.org/10.1016/j.matlet.2017.01.03. View Article
[11] Amaveda, H., Madre, M.A., Mora, M., Torres M. A., Sotelo A. Anomalous grain growth in sintered Bi2Ca2Co2−xCuxOy + Ag ceramic composites by Cu doping. Journal of Materials Science: Materials in Electronics. 34, 9, 2023. https://doi.org/10.1007/s10854-022-09416-x. View Article
[12] Margiani, N.; Zhghamadze, V.; Mumladze, G.; Takeda, M.; Kvartskhava, I. Thermoelectric properties of BiBO3-added Bi2Sr2Co2Oy ceramics. Journal of the Ceramic Society of Japan. 2025, in press.
[13] J. M. Nedelec, L. Courtheoux, E. Jallot, C. Kinowski, J. Lao, P. Laquerriere, C. Mansuy, G. Renaudin, S. Turrell. Materials Doping Through Sol–Gel Chemistry: A Little Something Can Make a Big Difference. Journal of Sol-Gel Science and Technology. 2008 46, 259–271. https://doi.org/10.1007/s10971-007-1665-0. View Article
[14] S. Rasekh, G. Constantinescu, M. A. Madre, M. Torres, J. Diez, A. Sotelo. Processing effects on the thermoelectric properties of Bi2Ca2Co1.7Ox ceramics. 2014, Boletin de la Sociedad Espanola de Ceramica y Vidrio. 53, 207-212. https://api.semanticscholar.org/CorpusID:204009217. View Article
[15] Rubešová, K.; Hlásek, T.; Jakeš, V.; Huber, Š.; Hejtmánek, J.; Sedmidubský, D. Effect of a powder compaction process on the thermoelectric properties of Bi2Sr2Co1.8Ox ceramics. Journal of the European Ceramic Society. 2015, 35, 525-531. DOI: 10.1016/j.jeurceramsoc.2014.08.037. View Article
[16] K. Rubešová, T. Hlásek, V. Jakeš, Š. Huber, J. Hejtmánek, D. Sedmidubský, Water based sol–gel methods used for Bi-222 thermoelectrics preparation. Journal of Sol-Gel Science and Technology. 64, 93-99, 2012. DOI 10.1007/s10971-012-2831-6. View Article
[17] Torres, M.A.; Sotelo, A.; Rasekh, Sh.; Serrano, I.; Constantinescu, G.; Madre, M.A.; Diez, J.C. Mejora de Las Propiedades Termoeléctricas de Bi2Sr2Co1.8Ox Por Métodos de Síntesis En Disolución. Boletin de la Sociedad Espanola de Ceramica y Vidrio. 2012, 51, 1–6. View Article
[18] A. Sotelo, Sh. Rasekh, M.A. Madre, E. Guilmeau, S. Marinel, J.C. Diez. Solution-based synthesis routes to thermoelectric Bi2Ca2Co1.7Ox, Journal of the European Ceramic Society. 2011, 31, 1763-1769. https://doi.org/10.1016/j.jeurceramsoc.2011.03.008. View Article
[19] Muguerra H, Rivas-Murias B, Traianidis M, Henrist C, Vertruyen B, Cloots R. Improvement of the thermoelectric properties of [Bi1.68Ca2O4-_d]RS[CoO2]1.69 cobaltite by chimie douce methods. Journal of solid state chemistry. 2010, 183:1252–7. https://doi.org/10.1016/j.jssc.2010.03.030. View Article
[20] Hao, H. S., He, Q. L., & Zhao, L. M. 2011, Thermoelectric Properties of Cu-Substituted Bi2Ca2Co2Oy Misfit Oxides. Advanced Materials Research, 284–286, 2263–2267. https://doi.org/10.4028/www.scientific.net/amr.284-286.2263. View Article