Volume 11 - Year 2024 - Pages 434-442
DOI: 10.11159/jffhmt.2024.043
Surroundings Impact on Terpene Stability in Terpene-Infused Pre-Rolled Cones: The Role of Temperature, Humidity, and Light Exposure
Arthur Karangan1,2†*, Antonius Jimmy Widagdo3,2†, Sherwin Wardhana Rahardjo1, Michael Giovanni Sugiarto3, Reinhard Ferdinand Christanto3, Edward Hartman Ernest3,2, Shella Permatasari Santoso3*, Suryadi Ismadji3
1Research and Development Department, PT. Mitra Prodin, Jl. Prof. Dr. Ida Bagus Mantra, Ketewel, Kecamatan Sukawati, Kabupaten Gianyar, Bali 80582, Indonesia
arthur.karangan@mitraprodin.com (A.K.), Sherwin.rahardjo@mitraprodin.com (S.W.R)
2Engineer Profession Education, Faculty of Engineering, Widya Mandala Surabaya Catholic University, Jl. Kalijudan No. 37, Surabaya 60114, East Java, Indonesia
3Chemical Engineering Department, Faculty of Engineering, Widya Mandala Surabaya Catholic University, Jl. Kalijudan No. 37, Surabaya 60114, East Java, Indonesia
liemchunhan1@gmail.com (A.J.W.), michaelgiovanni323@gmail.com (M.G.S.), reinfc02@gmail.com (R.F.C.), edwa.hartman@gmail.com (E.H.E.), shella@ukwms.ac.id (S.P.S.), suryadiismadji@yahoo.com (S.I.)
†These authors are equally contributed to this work
*Correspondence: arthur.karangan@mitraprodin.com (A.K.), shella@ukwms.ac.id (S.P.S.)
Abstract - Infusion of terpenes into the pre-rolled cone can enhance aroma and flavor and give the consumer a relaxing feeling. The high volatility of terpenes is a significant challenge in maintaining their content before reaching consumers. The terpene content can be lost due to evaporation during the product storage due to various environmental factors ―This study demonstrates the extent to which temperature (at 30–50°C), light intensity (at 200–1500 lux), and room humidity affect terpene evaporation rate. The results of the study showed that light exposure had the most significant effect on the rate of terpene evaporation, followed by temperature. Light exposure at 1200 lux for 24 h leads to 99% terpenes loss. Terpene degradation significantly increases with rising temperatures and reaches 92.5% at 50°C. Contrariwise, higher humidity levels were found to reduce the degradation rates. These findings provide valuable insights for industries to develop more stable and high-quality terpene-containing products.
Keywords: Terpene-infused cone, Terpene degradation, Terpene evaporation, Terpene stability.
© Copyright 2024 Authors - This is an Open Access article published under the Creative Commons Attribution License terms. Unrestricted use, distribution, and reproduction in any medium are permitted, provided the original work is properly cited.
Date Received: 2024-10-22
Date Revised: 2024-11-11
Date Accepted: 2024-12-03
Date Published: 2024-12-27
1. Introduction
The health risks of tobacco smoking are widely known and extensively documented. Every year, second-hand smoke is thought to be responsible for the deaths of nearly 8 million people, either from inhaling or exhaling cigarette vapor [1-3]. Innovative methods for producing "healthier" cigarettes have emerged as a result of growing awareness of the danger smoking poses to public health. This strategy can lessen the risk associated with tobacco use, particularly for smokers who are having trouble quitting their addiction [4]. Herbal (tobacco-free) cigarettes have emerged as a healthier alternative to tobacco cigarettes, although the comparative health dangers of herbal and tobacco cigarettes are still up for dispute [5, 6]. Herbal cigarettes are made with fillers from herbal plants and wrapped using pre-rolled cone paper [2]. Flavor infusion into a pre-rolled cone is often done to increase the user's relaxation sensation [7, 8]. Terpene is one of the infusion fluids that are in demand because it is said to provide a soothing effect [9, 10], but it also has benefits such as anticancer [11], antimicrobial [12], immune-modulatory, and anti-inflammatory activity [13]. Terpenes are a diverse group of plant organic compounds contributing to distinctive flavors and aromas [14]. Cannabis is the most popular plant for extracting terpenes [15-17]. Infusion of terpenes into pre-rolled cones can provide a variety of flavors, such as sweet, citrus, bitter, and pine. However, the production process of terpene-infused pre-rolled cones faces significant challenges, mainly related to the high volatility property of terpenes [18].
During production, terpenes can evaporate quickly, leading to inconsistent flavor profiles and reduced efficacy of the final product [7]. This volatility is exacerbated by fluctuations in environmental conditions, making it difficult for manufacturers to maintain the desired terpene concentration within the cones. This issue impacts product quality and increases production costs due to the need for additional terpene infusion to compensate for losses. Understanding the impact of environmental factors, such as humidity, temperature, and light exposure, on terpene stability is crucial for addressing these challenges. Environmental conditions can significantly influence the rate at which terpenes evaporate or degrade [19, 20], affecting the overall quality and consumer experience of terpene-infused pre-rolled cones. For instance, high temperatures can accelerate the evaporation of terpenes, while excessive humidity may lead to unwanted chemical reactions [21-23]. Additionally, light exposure, remarkably UV light, can cause terpene degradation and result in the loss of crucial aromatic compounds. Therefore, it is imperative to investigate how these environmental factors interact with terpene-infused pre-rolled cones to develop adequate storage and handling guidelines. By gaining a deeper understanding of these influences, manufacturers can optimize production processes and packaging solutions to preserve terpene-infused products' integrity and therapeutic efficacy, ensuring consumers' consistent and high-quality experience.
2. Methodology
2. 1. Materials and Chemicals
Cali Terpenes, Spain, provided terpene infusion fluid (99% purity). Pre-rolled cone paper with specifications, as described in Table 1, was provided by PT. Mitra Prodin (Bali, Indonesia). Polypropylene glycol (PPG) was obtained from Dow Chemical (Thailand). Ethanol (96% purity), chloroform (CHCl₃, 99% purity), and sulphuric acid (H₂SO₄, 98% purity) were obtained from Honeywell (Indonesia).
Table 1. The specification of pre-rolled cone paper used for terpene infusion
Parameter |
Unit |
Target |
Measured value |
Test method |
Grammage |
g/m2 |
14.0 |
13.0–15.0 |
ISO 536 |
Thickness |
μm |
27.0 |
Typical value |
ISO 534 |
Tensile |
N/15 mm |
16.0 |
13.0 (min.) |
ISO 1924-2 |
Stretch at break |
% |
1.3 |
1.1 (min.) |
ISO 1924-2 |
Opacity |
% |
48.0 |
52.0 (max.) |
ISO 2471 |
Brightness |
% |
20.0 |
15.0 (min.) |
ISO2470 |
Air permeability |
CU |
5 |
15.0 (max.) |
ISO 2965 |
Fiber furnish |
Wood |
|
|
|
2. 2. Terpene infusion into pre-rolled cone paper
Terpene infusion into the pre-rolled cone paper followed the standard production protocol applied at PT. Mitra Prodin (Bali, Indonesia) as described by Karangan, Rahardjo [7]. An equivolume of PPG, ethanol, and terpenes was mixed in a glass vial and vortexed for 10 seconds to ensure homogeneity. The pre-rolled cone paper was placed into a screw-capped borosilicate test tube (Qingdao Giant Packaging, China) ―The tube cap was made of polypropylene and equipped with a storage to inject the terpene-solution mixture. The schematic diagram and dimensions of the test tube are shown in Figure 1. After the terpene-solution mixture was injected into the cap, the cap was put onto the tube containing pre-rolled cone paper and heated at 80℃ in an oven (UN55, Memmert, Germany) for 60 mins. Then, the tube was removed from the oven and allowed to cool to room temperature overnight, with the cap remaining attached.
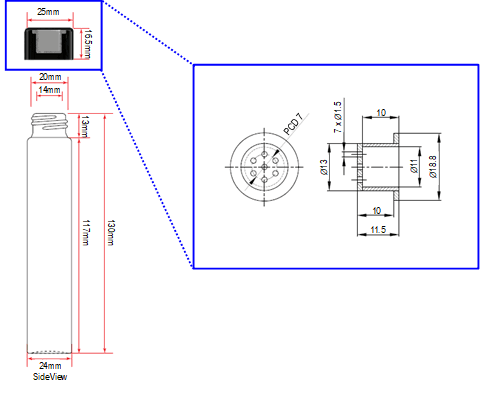
2. 3. Evaluation of the storage environment conditions to terpene loss
Effect of temperature. To quantify the effect of varying temperatures on terpene loss due to evaporation, the terpene-infused pre-rolled cone paper was incubated at different temperatures set at 30, 35, 40, 45, and 50°C. The incubation was carried out for 24 h in the dark.
Effect of humidity. To assess the impact of humidity, the terpene-infused pre-rolled cone paper was placed in an air-conditioned room with temperatures set at 26, 19, and 10°C. The incubation was carried out for 24 h in the dark.
Effect of light exposure. To investigate the effect of light exposure, the terpene-infused pre-rolled cone paper was placed in a device with light intensity variations of 200, 300, 600, 900, 1200, and 1500 lux for 24 h.
2. 4. Determination of terpene content
Standard curve preparation. Terpene content was estimated using a spectrophotometric procedure [7]. Briefly, a standard curve was prepared from terpene (99% purity, Cali Terpenes, Spain) at 10–90 μL volumes. Then, 1.5 mL of chloroform was added and vortexed for 10 s. Subsequently, 100 μL of concentrated H₂SO₄ was added to each mixture, and the samples were incubated in the dark for 1.5 h, leading to the formation of a reddish-brown precipitate. The supernatant was taken without disturbing the precipitate, and the precipitate was subsequently dissolved in 2.7 mL of 95% methanol. The mixture was vigorously shaken to form a homogeneous solution and then analyzed using a UV-Vis spectrophotometer (Genesys 150, Thermo Fisher Scientific, USA) at a wavelength of 538 nm.
Sample analysis. The terpene-infused pre-rolled cone paper was placed into a 5 mL test tube. 1.5 mL of chloroform was added, and the mixture was vortexed for 3 minutes to extract the terpene. The chloroform solution was then decanted, and subsequently, 100 μL of concentrated H₂SO₄ was added. The formed reddish-brown precipitate was analyzed using a spectrophotometric procedure as described previously.
3. Results and Discussion
3.1. Reaction mechanism in determination of terpene content
A straightforward and reliable technique for determining the amount of terpenes in a product containing terpenes was demonstrated in our earlier study [7]. The Salkowski test was used to detect terpenoids, which was the basis for developing the method [20]. In this procedure, terpene content in the terpene-containing product was extracted using chloroform. The reaction sequence of terpenes with sulfuric acid to produce precipitate is shown in Figure 2a:
- Acidic environment is created by adding concentrated H2SO4. The proton (H+) of H2SO4 induce the protonation of the terpene molecules. During this state, the precipitation does not yet form.
- The protonation of terpenes causes the double bonds in the terpene ring to break down, leading to carbo-cation formation (carbocation) as the intermediate product of the reaction.
- The carbocation subsequently reacts with sulfate ions (HSO₄⁺), forming a complex of alkyl sulfate compound. These alkyl sulfate complexes are large molecules with reduced solubility in chloroform/methanol solvent, forming a reddish-brown color precipitate with maximum absorbance at a visible wavelength (i.e., 538 nm).
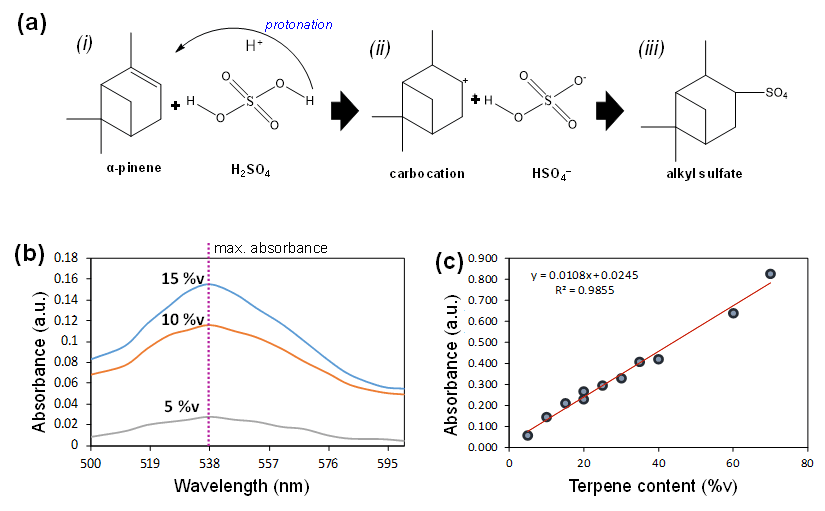
Figure 2b displays the maximum absorbance of the terpene precipitates in the visible wavelength range of 500–600 nm, as determined by a spectrophotometric technique. At various volumes of terpene addition (i.e., varied concentrations), the maximum absorbance of the precipitate consistently occurred at 538 nm. Repeated measurements showed minimal variation, with a discrepancy of ±2 nm. The linear relationship between absorbance and terpene content (%v) is shown in Figure 2c, as indicated by the R2 value close to unity (i.e., R2 = 0.9855). A linear correlation might also be observed for higher terpene concentrations (>70 %v). However, a standard curve was not created for these higher concentrations, as the actual product (infused pre-rolled cone paper) typically contains no more than ~30 %v terpene. A satisfactory correlation coefficient was obtained, demonstrating the applicability of the employed linear model.
3.2. Terpenes loss profile as an effect of surrounding conditions
In this study, the terpene content infused in the pre-rolled cone is being measured. During incubation, the terpene-infused pre-rolled cone paper was kept inside the glass tube with the cap open to amplify the effect of surrounding conditions. Subsequently, chloroform is used to extract the terpene content from the pre-rolled cone paper, and the spectrophotometric method is used for analysis (as previously discussed). Terpene content is decreased due to evaporating off the pre-rolled cone paper.
Figure 3a shows the reduction in terpene content due to evaporation from the pre-rolled cones following a 24 h incubation period at temperatures between 30 and 50°C. The calculated terpene loss rate is provided in Figure 3b. The findings conclusively demonstrate that terpene concentration decreases more rapidly as temperatures elevate. Terpene decreased from an initial content of 30.6%v to 20.5±0.2%v at the lowest investigated temperature of 30°C, with a calculated loss rate of 33.3±0.2%. At the maximum tested temperature of 50°C, a noticeably higher loss rate of 92.5±0.2% was recorded, and the amount of terpenes remaining in the pre-rolled cone paper was only 2.3±0.2%v. The loss of terpene content from the pre-rolled cone paper was primarily due to the evaporation of the compounds, as terpenes can begin to evaporate as low as 20°C [24]. At higher temperatures, a greater loss of terpenes and a lower terpene retention rate can be expected due to the increased rate of evaporation induced by higher thermal energy. Terpene loss due to degradation or compound breakdown was not observed in this case, as terpene degradation generally occurs at temperatures of 100°C or higher [25]. This finding suggests that low-temperature storage is better since it can slow down terpene loss.
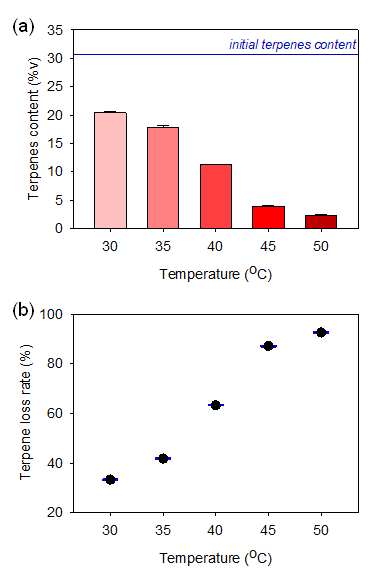
The light intensity was measured as the luminous flux per unit area (lux). As shown in Figure 4a and 4b, for the respective terpene level and loss rate, direct illumination on the terpene infused in pre-rolled cone paper can result in significant terpene content loss. At an illumination level of 200 lux, a high terpene degradation rate of 82.0% was recorded, which is close to the effect of terpene evaporation due to increased temperature. It is evident that terpene degradation rates were higher at greater illumination levels. At 1500 lux, it was found that ~100% of the terpene was being lost. The direct illumination on the terpenes may induce photo-degradation. In this context, the terpene degradation occurred due to the oxidation by hydroxyl radicals (OH•) generated from the photo-dissociation of water molecules from surrounding humidity [26]. Moreover, a direct correlation exists between higher lux levels and increasing surrounding temperature [27], with the latter increasing significantly as lux increases. Consequently, the rise in the temperature of the surroundings is also responsible for the significant amount of terpene loss at high levels of lux. It is evident from this result that dark conditions are preferable for preserving the quality of terpenes-containing products.
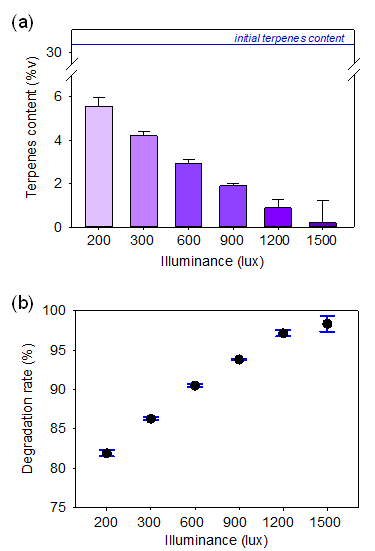
Figure 5a and 5b respectively shows the terpene content and loss rate as the effect of different relative humidity levels of surroundings. The result indicates an inverse correlation between humidity and terpene content loss, with higher humidity resulting in lower terpene loss. This phenomenon can be attributed to the hygroscopic property of pre-rolled cone paper, which absorbs moisture from the surrounding environment. The absorbed water molecules create a layer on the paper's surface, acting as a physical barrier preventing terpenes' evaporation. This result indicates that storing terpenes-containing products is more effective under humid conditions.
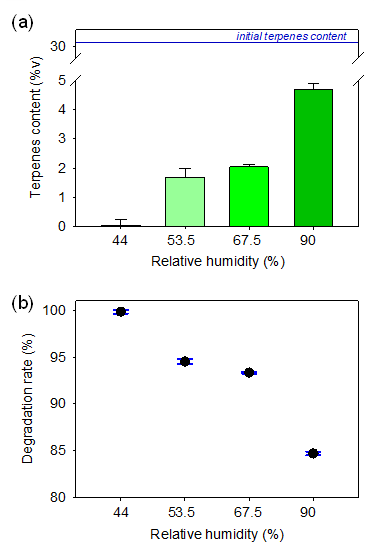
Figure 6 compares the terpene loss pathways from pre-rolled cone paper in open and closed systems. More terpenes will undoubtedly be lost to the surroundings with an open system. Meanwhile, the terpenes released from the paper circulate within the tube in a closed system and are not simply lost to the surroundings. As published in our study [7], The terpene content in the closed system increased with the incubation time as the terpenes were re-condensed and reabsorbed onto the paper.
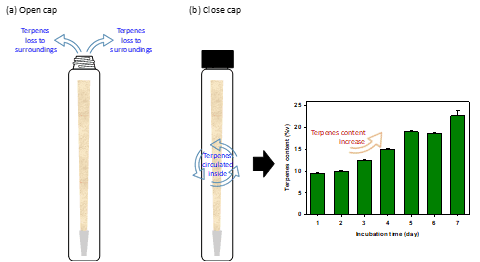
3. 3. Degradation mechanism of terpenes
Surrounding conditions impact the terpene content loss from pre-rolled cone paper. Terpene content loss primarily occurs due to evaporation since terpenes are inherently volatile. This study has demonstrated that the change in surrounding conditions influences the degradation rate of terpenes, as specific surrounding factors can initiate terpene oxidation and lead to their degradation. Direct light exposure to terpenes triggers the formation of hydroperoxide radicals, which can cause oxidation [28, 29]. Exposure of terpenes, such as limonene and linalool, to air can lead to the autoxidation of the compounds and forming hydroperoxide [30], and elevated temperature can cause the degradation to become more rapid [31].
Figure 7 illustrates the degradation pathway of a monoterpene, α-pinene, involving peroxy-radicals (RO2) [32], hydroperoxide (TERPOOH) [26], and organic nitrates (TERPNT) [33]. The degradation process begins with the reaction between α-pinene with hydroxyl radicals (•OH) forming from photo-oxidation of air moisture; this reaction produces peroxy-radicals (RO2) through the addition of oxygen molecules (O2), resulting in α-pinene-RO2. The α-pinene-RO2 molecules are reactive intermediates that can interact with various other radicals in the atmosphere and play a crucial role in subsequent reactions, forming various oxidation products.
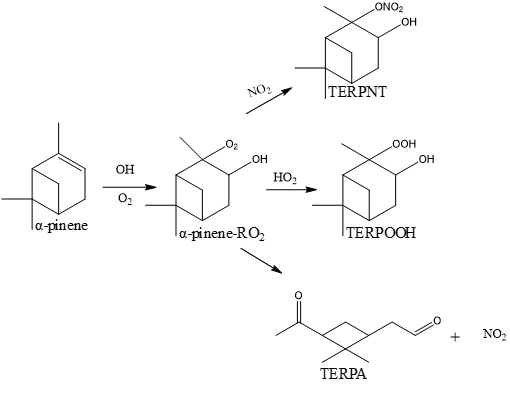
For instance, α-pinene-RO2 can react with NO2 to form an organic nitrate compound known as TERPNT, which contains a nitrate group (ONO2) attached to the terpene structure. Alternatively, α-pinene-RO2 can react with hydroperoxyl radicals (HO2) to form a hydroperoxide known as TERPOOH, which contains a hydroperoxide group (OOH) attached to the terpene structure. α-pinene-RO2 may also undergo decomposition or further reactions, resulting in end products such as TERPA pinon-aldehyde, involving ring opening and forming a carbonyl group (C=O).
TERPNT contains a nitrate group (ONO2) that makes it more reactive and contributes to the formation of aerosol particles in the atmosphere, while TERPOOH contains a hydroperoxide group (OOH), which is also highly reactive and can participate in aerosol particle formation. Pinon-aldehyde (TERPA) is an end product of the further reaction or decomposition of peroxy radicals, containing a carbonyl group (C=O) and acting as a volatile compound that can affect air quality. Overall, the degradation mechanism of α-pinene involves a series of complex oxidation reactions in the atmosphere, resulting in various oxidation products such as peroxy radicals, organic nitrates, hydroperoxide, and aldehydes.
Conclusion
Numerous studies have reported how environmental factors affect terpene loss due to evaporation or degradation. However, these studies are still limited to terpenes in their pure form. There is still no study investigating the loss of terpenes in the form of consumer goods, i.e., terpenes-infused pre-rolled cone papers. Limited documentation on the impact of external factors on terpene loss from infused rolling papers poses a challenge for the industry in developing appropriate storage to maintain the high quality of terpene-containing goods. The current study summarized how environmental conditions affect the terpene content loss during storage, including temperature, humidity, and illumination. While higher storage temperatures can accelerate the evaporation of terpenes, air humidity and light exposure may induce free radicals that can cause terpenes degradation. These insights highlight the importance of controlling environmental conditions to preserve terpene stability and improve the sensory qualities of the products.
Acknowledgments
This work was financially supported by PT. Mitra Prodin under agreement No. 010/LEG-MP/ADD/III/2024.
References
[1] Statista, Smoking worldwide - Statistics & Facts. 2024, Statista: Statista Research Department.
[2] R.T.A. Rahman, N. Kamal, A. Mediani, M.A. Farag, How Do Herbal Cigarettes Compare To Tobacco? A Comprehensive Review of Their Sensory Characters, Phytochemicals, and Functional Properties, ACS Omega 7 (2022) 45797-45809. https://doi.org/10.1021/acsomega.2c04708.
View Article
[3] R. West, Tobacco smoking: Health impact, prevalence, correlates and interventions, Psychology & Health 32 (2017) 1018-1036. https://doi.org/10.1080/08870446.2017.1325890.
View Article
[4] S.A. Baig, M.J. Byron, A.J. Lazard, N.T. Brewer, "Organic," "Natural," and "Additive-Free" Cigarettes: Comparing the Effects of Advertising Claims and Disclaimers on Perceptions of Harm, Nicotine & Tobacco Research 21 (2019) 933-939. https://doi.org/10.1093%2Fntr%2Fnty036.
View Article
[5] J.R. Sanchez-Ramos, The rise and fall of tobacco as a botanical medicine, Journal of Herbal Medicine 22 (2020) 100374. https://doi.org/10.1016%2Fj.hermed.2020.100374.
View Article
[6] J.H. Bak, S.M. Lee, H.B. Lim, Safety Assessment of Mainstream Smoke of Herbal Cigarette, Toxicological Research 31 (2015) 41-48. https://doi.org/10.5487%2FTR.2015.31.1.041.
View Article
[7] A. Karangan, S.W. Rahardjo, A.J. Widagdo, S.P. Santoso, S. Ismadji, Data on terpene content in pre-rolled cone paper infused with terpene-containing flavours based on the formation of a partially soluble precipitate, Data in Brief 55 (2024) 110623. https://doi.org/10.1016/j.dib.2024.110623.
View Article
[8] J. Bueno, E. Leuer, M. Kearney, E.H. Green, E.A. Greenbaum, The preservation and augmentation of volatile terpenes in cannabis inflorescence, Journal of Cannabis Research 2 (2020) 27. https://doi.org/10.1186%2Fs42238-020-00035-z.
View Article
[9] J.E. LaVigne, R. Hecksel, A. Keresztes, J.M. Streicher, Cannabis sativa terpenes are cannabimimetic and selectively enhance cannabinoid activity, Scientific Reports 11 (2021) 8232. https://doi.org/10.1038/s41598-021-87740-8.
View Article
[10] S.G. Ferber, D. Namdar, D. Hen-Shoval, G. Eger, H. Koltai, G. Shoval, L. Shbiro, A. Weller, The "Entourage Effect": Terpenes Coupled with Cannabinoids for the Treatment of Mood Disorders and Anxiety Disorders, Current Neuropharmacology 18 (2020) 87-96. https://doi.org/10.2174%2F1570159X17666190903103923.
View Article
[11] P. Wróblewska-Łuczka, J. Cabaj, J. Bargieł, J.J. Łuszczki, Anticancer effect of terpenes: focus on malignant melanoma, Pharmacological Reports 75 (2023) 1115-1125. https://doi.org/10.1007%2Fs43440-023-00512-1.
View Article
[12] N.A. Mahizan, S.-K. Yang, C.-L. Moo, A.A.-L. Song, C.-M. Chong, C.-W. Chong, A. Abushelaibi, S.-H.E. Lim, K.-S. Lai, Terpene Derivatives as a Potential Agent against Antimicrobial Resistance (AMR) Pathogens, Molecules 24 (2019) 2631. https://doi.org/10.3390%2Fmolecules24142631.
View Article
[13] M.L.D. Prado-Audelo, H. Cortés, I.H. Caballero-Florán, M. González-Torres, L. Escutia-Guadarrama, S.A. Bernal-Chávez, D.M. Giraldo-Gomez, J.J. Magaña, G. Leyva-Gómez, Therapeutic Applications of Terpenes on Inflammatory Diseases, Frontiers in Pharmacology 12 (2021) 704197. https://doi.org/10.3389%2Ffphar.2021.704197.
View Article
[14] A. Masyita, R.M. Sari, A.D. Astuti, B. Yasir, N.R. Rumata, T.B. Emran, F. Nainu, J. Simal-Gandara, Terpenes and terpenoids as main bioactive compounds of essential oils, their roles in human health and potential application as natural food preservatives, Food Chemistry: X 13 (2022) 100217. https://doi.org/10.1016%2Fj.fochx.2022.100217.
View Article
[15] M.M. Radwan, S. Chandra, S. Gul, M.A. ElSohly, Cannabinoids, Phenolics, Terpenes and Alkaloids of Cannabis, Molecules 26 (2021) 2774. https://doi.org/10.3390%2Fmolecules26092774.
View Article
[16] S.R. Sommano, C. Chittasupho, W. Ruksiriwanich, P. Jantrawut, The Cannabis Terpenes, Molecules 25 (2020) 5792. https://doi.org/10.3390%2Fmolecules25245792.
View Article
[17] J.K. Booth, J. Bohlmann, Terpenes in Cannabis sativa - From plant genome to humans, Plant Science 284 (2019) 67-72. https://doi.org/10.1016/j.plantsci.2019.03.022.
View Article
[18] T.-D. Nguyen, S. Riordan-Short, T.-T.T. Dang, R. O'Brien, M. Noestheden, Quantitation of Select Terpenes/Terpenoids and Nicotine Using Gas Chromatography-Mass Spectrometry with High-Temperature Headspace Sampling, ACS Omega 5 (2020) 5565-5573. https://doi.org/10.1021/acsomega.0c00384.
View Article
[19] V. Ninkuu, L. Zhang, J. Yan, Z. Fu, T. Yang, H. Zeng, Biochemistry of Terpenes and Recent Advances in Plant Protection, International Journal of Molecular Sciences 22 (2021) 5710. https://doi.org/10.3390%2Fijms22115710.
View Article
[20] Z. Jiang, C. Kempinski, J. Chappell, Extraction and Analysis of Terpenes/Terpenoids, Current Protocols in Plant Biology 1 (2016) 345-358. https://doi.org/10.1002%2Fcppb.20024.
View Article
[21] I. Poulopoulou, I. Hadjigeorgiou, Evaluation of Terpenes' Degradation Rates by Rumen Fluid of Adapted and Non-adapted Animals, Natural Products and Bioprospecting 11 (2021) 307-313. https://doi.org/10.1007%2Fs13659-020-00289-3.
View Article
[22] M. Lubinska-Szczygel, Ż. Polkowska, B. Tobolkova, T. Majchrzak, M. Polovka, P. Promchote, S. Gorinstein, Evaluation of Terpene Decomposition in Kaffir Lime Juice during Storage Using Gas Chromatography-Mass Spectrometry and Proton Transfer Reaction-Mass Spectrometry, Molecules 29 (2024) 3241. https://doi.org/10.3390/molecules29133241.
View Article
[23] N. Mokrani, T. Fateh, L. Courty, Thermal degradation of α-pinene and β-pinene: An experimental study, Fuel 267 (2020) 117177. https://doi.org/10.1016/j.fuel.2020.117177.
View Article
[25] Y. Yang, B. Kayan, N. Bozer, B. Pate, C. Baker, A.M. Gizir, Terpene degradation and extraction from basil and oregano leaves using subcritical water, Journal of Chromatography A 1152 (2007) 262-267. https://doi.org/10.1016/j.chroma.2006.11.037.
View Article
[26] R.H. Schwantes, L.K. Emmons, J.J. Orlando, M.C. Barth, G.S. Tyndall, S.R. Hall, K. Ullmann, J.M.S. Clair, D.R. Blake, A. Wisthaler, T.P.V. Bui, Comprehensive isoprene and terpene gas-phase chemistry improves simulated surface ozone in the southeastern US, Atmospheric Chemistry and Physics 20 (2020) 3739-3776. https://doi.org/10.5194/acp-20-3739-2020.
View Article
[27] M.t. Kulve, L. Schlangen, W.v.M. Lichtenbelt, Interactions between the perception of light and temperature, Indoor Air 28 (2018) 881-891. https://doi.org/10.1111/ina.12500.
View Article
[28] H. Bitterling, P. Lorenz, W. Vetter, D.R. Kammerer, F.C. Stintzing, Photo-protective effects of selected furocoumarins on β-pinene, R-(+)-limonene and γ-terpinene upon UV-A irradiation, Journal of Photochemistry and Photobiology A: Chemistry 424 (2022) 113623. https://doi.org/10.1016/j.jphotochem.2021.113623.
View Article
[29] F. Li, I.D. Boateng, S. Chen, X.-M. Yang, D.A. Soetanto, W. Liu, Pulsed light irradiation improves degradation of ginkgolic acids and retainment of ginkgo flavonoids and terpene trilactones in Ginkgo biloba leaves, Industrial Crops and Products 204 (2023) 117297. https://doi.org/10.1016/j.indcrop.2023.117297.
View Article
[30] A. Natsch, M. Nägelin, H. Leijs, M.v. Strien, E. Giménez-Arnau, M. Vey, C. González, Exposure source for skin sensitizing hydroperoxides of limonene and linalool remains elusive: An analytical market surveillance, Food and Chemical Toxicology 127 (2019) 156-162. https://doi.org/10.1016/j.fct.2019.03.028.
View Article
[31] F. Guerrero, C. Carmona, C. Hernández, M. Toledo, A. Arriagada, L. Espinoza, J. Bergmann, L. Taborga, K. Yañez, Y. Carrasco, A.A. Muñoz, Drivers of Flammability of Eucalyptus globulus Labill Leaves: Terpenes, Essential Oils, and Moisture Content, Forests 13 (2022) 908. https://doi.org/10.3390/f13060908.
View Article
[32] S. Richters, H. Herrmann, T. Berndt, Highly Oxidized RO2 Radicals and Consecutive Products from the Ozonolysis of Three Sesquiterpenes, Environmental Science & Technology 50 (2016) 2354-2362. https://doi.org/10.1021/acs.est.5b05321.
View Article
[33] J.H. Slade, C.d. Perre, L. Lee, P.B. Shepson, Nitrate radical oxidation of-terpinene: hydroxy nitrate, total organic nitrate, and secondary organic aerosol yields, Atmospheric Chemistry and Physics 17 (2017) 8635-8650. https://doi.org/10.5194/acp-17-8635-2017.
View Article
[1] Statista, Smoking worldwide - Statistics & Facts. 2024, Statista: Statista Research Department.
[2] R.T.A. Rahman, N. Kamal, A. Mediani, M.A. Farag, How Do Herbal Cigarettes Compare To Tobacco? A Comprehensive Review of Their Sensory Characters, Phytochemicals, and Functional Properties, ACS Omega 7 (2022) 45797-45809. https://doi.org/10.1021/acsomega.2c04708. View Article
[3] R. West, Tobacco smoking: Health impact, prevalence, correlates and interventions, Psychology & Health 32 (2017) 1018-1036. https://doi.org/10.1080/08870446.2017.1325890. View Article
[4] S.A. Baig, M.J. Byron, A.J. Lazard, N.T. Brewer, "Organic," "Natural," and "Additive-Free" Cigarettes: Comparing the Effects of Advertising Claims and Disclaimers on Perceptions of Harm, Nicotine & Tobacco Research 21 (2019) 933-939. https://doi.org/10.1093%2Fntr%2Fnty036. View Article
[5] J.R. Sanchez-Ramos, The rise and fall of tobacco as a botanical medicine, Journal of Herbal Medicine 22 (2020) 100374. https://doi.org/10.1016%2Fj.hermed.2020.100374. View Article
[6] J.H. Bak, S.M. Lee, H.B. Lim, Safety Assessment of Mainstream Smoke of Herbal Cigarette, Toxicological Research 31 (2015) 41-48. https://doi.org/10.5487%2FTR.2015.31.1.041. View Article
[7] A. Karangan, S.W. Rahardjo, A.J. Widagdo, S.P. Santoso, S. Ismadji, Data on terpene content in pre-rolled cone paper infused with terpene-containing flavours based on the formation of a partially soluble precipitate, Data in Brief 55 (2024) 110623. https://doi.org/10.1016/j.dib.2024.110623. View Article
[8] J. Bueno, E. Leuer, M. Kearney, E.H. Green, E.A. Greenbaum, The preservation and augmentation of volatile terpenes in cannabis inflorescence, Journal of Cannabis Research 2 (2020) 27. https://doi.org/10.1186%2Fs42238-020-00035-z. View Article
[9] J.E. LaVigne, R. Hecksel, A. Keresztes, J.M. Streicher, Cannabis sativa terpenes are cannabimimetic and selectively enhance cannabinoid activity, Scientific Reports 11 (2021) 8232. https://doi.org/10.1038/s41598-021-87740-8. View Article
[10] S.G. Ferber, D. Namdar, D. Hen-Shoval, G. Eger, H. Koltai, G. Shoval, L. Shbiro, A. Weller, The "Entourage Effect": Terpenes Coupled with Cannabinoids for the Treatment of Mood Disorders and Anxiety Disorders, Current Neuropharmacology 18 (2020) 87-96. https://doi.org/10.2174%2F1570159X17666190903103923. View Article
[11] P. Wróblewska-Łuczka, J. Cabaj, J. Bargieł, J.J. Łuszczki, Anticancer effect of terpenes: focus on malignant melanoma, Pharmacological Reports 75 (2023) 1115-1125. https://doi.org/10.1007%2Fs43440-023-00512-1. View Article
[12] N.A. Mahizan, S.-K. Yang, C.-L. Moo, A.A.-L. Song, C.-M. Chong, C.-W. Chong, A. Abushelaibi, S.-H.E. Lim, K.-S. Lai, Terpene Derivatives as a Potential Agent against Antimicrobial Resistance (AMR) Pathogens, Molecules 24 (2019) 2631. https://doi.org/10.3390%2Fmolecules24142631. View Article
[13] M.L.D. Prado-Audelo, H. Cortés, I.H. Caballero-Florán, M. González-Torres, L. Escutia-Guadarrama, S.A. Bernal-Chávez, D.M. Giraldo-Gomez, J.J. Magaña, G. Leyva-Gómez, Therapeutic Applications of Terpenes on Inflammatory Diseases, Frontiers in Pharmacology 12 (2021) 704197. https://doi.org/10.3389%2Ffphar.2021.704197. View Article
[14] A. Masyita, R.M. Sari, A.D. Astuti, B. Yasir, N.R. Rumata, T.B. Emran, F. Nainu, J. Simal-Gandara, Terpenes and terpenoids as main bioactive compounds of essential oils, their roles in human health and potential application as natural food preservatives, Food Chemistry: X 13 (2022) 100217. https://doi.org/10.1016%2Fj.fochx.2022.100217. View Article
[15] M.M. Radwan, S. Chandra, S. Gul, M.A. ElSohly, Cannabinoids, Phenolics, Terpenes and Alkaloids of Cannabis, Molecules 26 (2021) 2774. https://doi.org/10.3390%2Fmolecules26092774. View Article
[16] S.R. Sommano, C. Chittasupho, W. Ruksiriwanich, P. Jantrawut, The Cannabis Terpenes, Molecules 25 (2020) 5792. https://doi.org/10.3390%2Fmolecules25245792. View Article
[17] J.K. Booth, J. Bohlmann, Terpenes in Cannabis sativa - From plant genome to humans, Plant Science 284 (2019) 67-72. https://doi.org/10.1016/j.plantsci.2019.03.022. View Article
[18] T.-D. Nguyen, S. Riordan-Short, T.-T.T. Dang, R. O'Brien, M. Noestheden, Quantitation of Select Terpenes/Terpenoids and Nicotine Using Gas Chromatography-Mass Spectrometry with High-Temperature Headspace Sampling, ACS Omega 5 (2020) 5565-5573. https://doi.org/10.1021/acsomega.0c00384. View Article
[19] V. Ninkuu, L. Zhang, J. Yan, Z. Fu, T. Yang, H. Zeng, Biochemistry of Terpenes and Recent Advances in Plant Protection, International Journal of Molecular Sciences 22 (2021) 5710. https://doi.org/10.3390%2Fijms22115710. View Article
[20] Z. Jiang, C. Kempinski, J. Chappell, Extraction and Analysis of Terpenes/Terpenoids, Current Protocols in Plant Biology 1 (2016) 345-358. https://doi.org/10.1002%2Fcppb.20024. View Article
[21] I. Poulopoulou, I. Hadjigeorgiou, Evaluation of Terpenes' Degradation Rates by Rumen Fluid of Adapted and Non-adapted Animals, Natural Products and Bioprospecting 11 (2021) 307-313. https://doi.org/10.1007%2Fs13659-020-00289-3. View Article
[22] M. Lubinska-Szczygel, Ż. Polkowska, B. Tobolkova, T. Majchrzak, M. Polovka, P. Promchote, S. Gorinstein, Evaluation of Terpene Decomposition in Kaffir Lime Juice during Storage Using Gas Chromatography-Mass Spectrometry and Proton Transfer Reaction-Mass Spectrometry, Molecules 29 (2024) 3241. https://doi.org/10.3390/molecules29133241. View Article
[23] N. Mokrani, T. Fateh, L. Courty, Thermal degradation of α-pinene and β-pinene: An experimental study, Fuel 267 (2020) 117177. https://doi.org/10.1016/j.fuel.2020.117177. View Article
[25] Y. Yang, B. Kayan, N. Bozer, B. Pate, C. Baker, A.M. Gizir, Terpene degradation and extraction from basil and oregano leaves using subcritical water, Journal of Chromatography A 1152 (2007) 262-267. https://doi.org/10.1016/j.chroma.2006.11.037. View Article
[26] R.H. Schwantes, L.K. Emmons, J.J. Orlando, M.C. Barth, G.S. Tyndall, S.R. Hall, K. Ullmann, J.M.S. Clair, D.R. Blake, A. Wisthaler, T.P.V. Bui, Comprehensive isoprene and terpene gas-phase chemistry improves simulated surface ozone in the southeastern US, Atmospheric Chemistry and Physics 20 (2020) 3739-3776. https://doi.org/10.5194/acp-20-3739-2020. View Article
[27] M.t. Kulve, L. Schlangen, W.v.M. Lichtenbelt, Interactions between the perception of light and temperature, Indoor Air 28 (2018) 881-891. https://doi.org/10.1111/ina.12500. View Article
[28] H. Bitterling, P. Lorenz, W. Vetter, D.R. Kammerer, F.C. Stintzing, Photo-protective effects of selected furocoumarins on β-pinene, R-(+)-limonene and γ-terpinene upon UV-A irradiation, Journal of Photochemistry and Photobiology A: Chemistry 424 (2022) 113623. https://doi.org/10.1016/j.jphotochem.2021.113623. View Article
[29] F. Li, I.D. Boateng, S. Chen, X.-M. Yang, D.A. Soetanto, W. Liu, Pulsed light irradiation improves degradation of ginkgolic acids and retainment of ginkgo flavonoids and terpene trilactones in Ginkgo biloba leaves, Industrial Crops and Products 204 (2023) 117297. https://doi.org/10.1016/j.indcrop.2023.117297. View Article
[30] A. Natsch, M. Nägelin, H. Leijs, M.v. Strien, E. Giménez-Arnau, M. Vey, C. González, Exposure source for skin sensitizing hydroperoxides of limonene and linalool remains elusive: An analytical market surveillance, Food and Chemical Toxicology 127 (2019) 156-162. https://doi.org/10.1016/j.fct.2019.03.028. View Article
[31] F. Guerrero, C. Carmona, C. Hernández, M. Toledo, A. Arriagada, L. Espinoza, J. Bergmann, L. Taborga, K. Yañez, Y. Carrasco, A.A. Muñoz, Drivers of Flammability of Eucalyptus globulus Labill Leaves: Terpenes, Essential Oils, and Moisture Content, Forests 13 (2022) 908. https://doi.org/10.3390/f13060908. View Article
[32] S. Richters, H. Herrmann, T. Berndt, Highly Oxidized RO2 Radicals and Consecutive Products from the Ozonolysis of Three Sesquiterpenes, Environmental Science & Technology 50 (2016) 2354-2362. https://doi.org/10.1021/acs.est.5b05321. View Article
[33] J.H. Slade, C.d. Perre, L. Lee, P.B. Shepson, Nitrate radical oxidation of-terpinene: hydroxy nitrate, total organic nitrate, and secondary organic aerosol yields, Atmospheric Chemistry and Physics 17 (2017) 8635-8650. https://doi.org/10.5194/acp-17-8635-2017. View Article