Volume 11 - Year 2024 - Pages 233-239
DOI: 10.11159/jffhmt.2024.023
Impact of Various Thermochromic Materials for Thermal Storage Applications on Multilayer Thin Film Coatings
Abdullatif Hakami1
1Department of Electrical Engineering, College of Engineering, University of Bisha, Bisha 61922, P.O. Box 551, Saudi Arabia.
abhakami@ub.edu.sa
Abstract - Solar radiation causes a remarkably high amount of thermal energy to be gained or lost in buildings. Appropriate window layout and design as well as intelligent roof coatings help a building maintain a suitable temperature. Applied to glass or roof surfaces, single- or multilayer thin film coatings with spectrally desired properties can significantly increase a building's energy efficiency. Thermochromic materials present some advantages due to their reversible color and phase change behavior near ambient temperature. For example, a thermochromic coating on a roof’s surface can change color (black to white) reversibly when heated at around 30 oC, reflecting solar radiation and reducing a building’s cooling needs. In the present study, various thermochromic coatings on glass slides are investigated. In this work, the effect of non-toxic titanium dioxide (TiO2), used as a UV radiation protective coating on thermochromic particles (such as a three-component blue dye synthesized in the lab or a commercially available black dye), is discussed. The composite coatings of the chosen components can reduce a building’s cooling and heating energy needs and provide an environmentally sustainable solution for a green economy. Several physico-chemical characterization techniques were used to understand the surface, interfacial, spectroscopic, and thermal behavior of TiO2 or SiO2 coatings and phase change thermochromic materials for applications in thermal storage and enhanced energy efficiency.
Keywords: Thermal energy, glass substrate, phase change, smart thermochromic coating, built environment.
© Copyright 2024 Authors - This is an Open Access article published under the Creative Commons Attribution License terms. Unrestricted use, distribution, and reproduction in any medium are permitted, provided the original work is properly cited.
Date Received: 2024-02-05
Date Revised: 2024-07-12
Date Accepted: 2024-07-24
Date Published: 2024-xx-xx
1. Introduction
In contemporary times, there has been a significant increase in the utilization of heating and cooling systems, which are imperative for the provision of optimal comfort levels within various architectural structures. This phenomenon results in a rise in power consumption and the release of greenhouse gas emissions [1]. In recent years, there are many important of amount of study which dedicated to investigating this particular subject [2] resulting in the development of novel technological strategies and mitigation of the phenomenon known as the "heat island" effect [3]. The utilization of various materials as smart coatings has a substantial influence on the thermal envelope of a building [4] Notably, smart windows and cool roofs are widely recognized as the most prominent applications in this case [5].
The utilization of diverse coatings to achieve fenestration with a high level of reflectivity is a prevalent practice in multiple construction applications, particularly in urban environments. Additionally, this feature contributes to the provision of optimal thermal conditions for outside area and their immediate vicinity [6]. Additionally, in this case of various layer of thin or thick film which coating on materials has unique possess appropriate as absorptive capacity, reflective surface, and transmissive power, thereby contributing to the promotion of energy conservation during periods of high temperatures [7]. Thermochromic smart materials has advantage as the potential to move a substantial position at mitigating a building's energy demands during periods of both high and low temperatures [8]. The implementation of intelligent thermochromic materials on the surfaces of external window glass can lead to a decrease in room temperature through the alteration of the glass surface color.
While windows only occupy a relatively small area of a building's exterior, the utilization of various thermochromic materials can yield substantial energy savings. Over the precent years, there are a gradual grow up in the utilization of thermos-chromic coatings with different kinds of film size on the roof of building and windows as well of buildings [9]. The current investigation utilized a substrate composed of a thick glass measuring 2 mm in thickness to accommodate the application of thermochromic coatings. The optical components of the coatings that have been developed are subjected to testing. This study utilized two types of dye: (1) a commercially on market find as black dye and (2) a lab-synthesized with other type of day as drak blue dye composed of three components. These dyes were chosen as thermochromic materials for the experiment. The selection of TiO2 or SiO2 was made in order to provide protection for polymer-based thermochromic materials against photodegradation or heat degradation.
A variety of thermochromic coatings were applied to glass surfaces with a thickness of 2 mm, resulting in the preparation of multiple samples. The experimental materials utilized in this study include powdered titanium dioxide (TiO2) with a minimum purity of 98.9%, silicon dioxide (SiO2) with a purity of 99%, which encapsulated for this kind of dark/white, blue dye synthesized through watery chemistry method, a three-component as dark/white, blue dye, and market findling as a dark black dye. The black dye was tested both with and without a second layer coating of TiO2. The investigation involved the combination of specific constituents with a sodium metasilicate which work likes binder for analysis of these parts. The 3-component mothed of this kind of day for the blue dye consisted of 1-tetra-decanol which used as main solvent, crystal-violet-lactone (C-V-L) as the primary dye techniques, and bi-sphenol A (B-P-A) which use it as main objective as color developer. The previous literature has documented the encapsulation process of the blue dye [10]. The utilization of the black dye encompassed both its chromatic alteration and its alteration in state upon reaching a temperature exceeding 30 degrees Celsius. Sodium silicate was employed as a binding agent in the thermochromic samples containing both black and blue dyes. Table 1 presents comprehensive information regarding the various components of the studied system, including their respective masses[11].
2. Experimental
The following are concise explanations outlining the procedures for preparing the precursor compounds used in the production of black and blue dyes. The primary composition (expressed in weight percent) of the forerunner utilized with a small glass by applying the day as coating about 1.50 wt%. The sodium silicate liquid film binder, with a volume ranging from 0.70 to 0.80 ml, was combined with water. The components were combined in a depth of the corner of bottom flask and agitated at a rate rotational speed of 800.0 revolutions per minute (r-p-m) for a duration of 2 hours. Subsequently, the appropriate quantity of pure TiO2 powder was introduced into the mixture and agitated for a duration of 45 minutes. Subsequently, the rotational velocity was decreased to 700 revolutions per minute (rpm), and a minute quantity, approximately 0.10%, of undiluted dark black/dark-blue dye was introduced into the amalgamation. Ultimately, a mixed solution that exhibited homogeneity and encompassed all constituent components was successfully acquired and subsequently employed for the purpose of coating. The act of stirring was consistently maintained throughout the entirety of the process.
The following are concise explanations outlining the procedures for preparing the precursor compounds used in the production of black and blue dyes. The primary composition (expressed in weight percent) of the forerunner utilized with a small glass by applying the day as coating about 1.50 wt%. The sodium silicate liquid film binder, with a volume ranging from 0.70 to 0.80 ml, was combined with water. The components were combined in a depth of the corner of bottom flask and agitated at a rate rotational speed of 800.0 revolutions per minute (r-p-m) for a duration of 2 hours. Subsequently, the appropriate quantity of pure TiO2 powder was introduced into the mixture and agitated for a duration of 45 minutes. Subsequently, the rotational velocity was decreased to 700 revolutions per minute (rpm), and a minute quantity, approximately 0.10%, of undiluted dark black/dark-blue dye was introduced into the amalgamation. Ultimately, a mixed solution that exhibited homogeneity and encompassed all constituent components was successfully acquired and subsequently employed for the purpose of coating. The act of stirring was consistently maintained throughout the entirety of the process.
The following are concise explanations outlining the procedures for preparing the precursor compounds used in the production of black and blue dyes. The primary composition (expressed in weight percent) of the forerunner utilized with a small glass by applying the day as coating about 1.50 wt%. The sodium silicate liquid film binder, with a volume ranging from 0.70 to 0.80 ml, was combined with water. The components were combined in a depth of the corner of bottom flask and agitated at a rate rotational speed of 800.0 revolutions per minute (r-p-m) for a duration of 2 hours. Subsequently, the appropriate quantity of pure TiO2 powder was introduced into the mixture and agitated for a duration of 45 minutes. Subsequently, the rotational velocity was decreased to 700 revolutions per minute (rpm), and a minute quantity, approximately 0.10%, of undiluted dark black/dark-blue dye was introduced into the amalgamation. Ultimately, a mixed solution that exhibited homogeneity and encompassed all constituent components was successfully acquired and subsequently employed for the purpose of coating. The act of stirring was consistently maintained throughout the entirety of the process.
This study involved the preparation of six different types of samples, as shown in Table 1. The glass substrate was placed within a film applicator, commonly known as a doctor blade, in order to achieve a consistent coating thickness of 300 µm. A visual representation of this process can be found in Figure 1A. The samples underwent a drying process at a temperature of 20°C, commonly referred to as room temperature, for a minimum duration of 6 hours. Adequate protection was provided to all samples through the use of an appropriate parafilm to cover it. The primary objective of using this kind of study is to investigate the efficacy of different sizes applied and coated on glass with dyes in terms of their performance behaviors. Various analytical tools, including a UV-Vis (illustrated in Fig 1B), Differential-Scanning-Calorimeter, Scanning-Electron-Microscope, and Energy-Dispersive x-ray detector, were utiliz to characterize these thin films. Many color coordinates for different samples were quantified using the CIE L*a*b* (International Commission on Illumination) system. In this system, the lightness index is represented by L*, while the several values indicated the green to red and blue to yellow which colors complexes are indicated by a* and b* respectively [12].
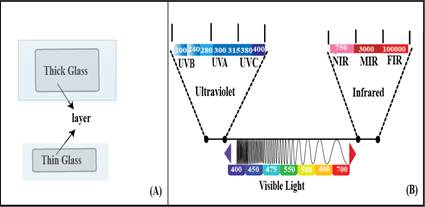
The weight percent (wt%) of the dye was determined by employing equation (1) as referenced in a previous study [13]

3. Results and Discussion
The optical spectra of the samples were acquired using a 5000 UV-Visible-NIR spectrophotometer. Analyzed were the spectra of the two colorful (20 oC) and clear films (30-40 oC) that were placed on 2mm glass. The ultraviolet-visible spectrum of all thin film materials indicated in Table 1 at 20 oC and 40 oC are depicted in Figure 2 (A-F). The samples under consideration consist of binder and CVL leuco dye-based black and blue dyes. These dyes are individually encapsulated by SiO2 and TiO2, with the exception of a single case where the black dye was not isolated by SiO2. In order to enhance the accuracy of the assessment of the binder-TiO2 coating, additional measurements were taken for its absorption, transmission, and reflected spectra. However, these spectra were not included in the analysis because to page constraints. In our study, we employed CVL as the leuco dye in the three-component system. However, it is necessary to validate the nature of the readily available black dye [14].
The absorption performance of the blue dye, black dye, and CVL was compared in a study conducted by K. Takaoka et al. This work, both theoretically and empirically, provided insights into the characteristics of black dye. CVL is characterized by the presence of colorless lactone rings. The lactone ring undergoes proton addition upon a temperature shift inside the system. Consequently, a modification in hue occurs, resulting in the manifestation of the thermochromic phenomenon.
Table 1: Various Constituents Utilizing a Binder.
Components of TCM |
Masses of dye components (g) |
Total mass of the solution determined by considering the combined masses (g) |
Puer black dye & binder |
13.080 |
17.03 |
Puer blue dye & binder |
12.850 |
15.01 |
TiO2 as powder & binder |
12.550 |
15.20 |
SiO2 & blue dye gel (wet chemistry) |
13.080 |
16.09 |
TiO2 & blue dye (layer coating) |
13.750 |
16.33 |
TiO2 & black dye (layer coating) |
14.080 |
16.90 |
At approximately 621 nm, 571 nm, and 471 nm, three distinct absorption bands are observable. The bands are attributed to π→π* changes of the aminophenyl rings of CVL, which take place from the highest occupied molecular orbital (HOMO) to lowest unoccupied molecular orbital (LUMO) levels, in accordance with the molecular orbital studies. The reversible opening and shutting of the CVL lactone loop in the case of a proton is depicted in Scheme 1.
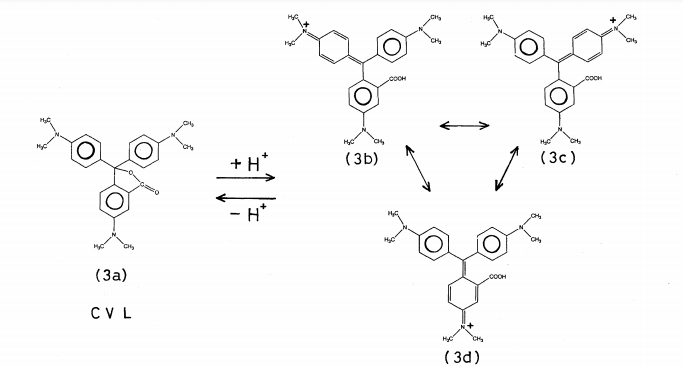
In Figure 2A, it can be observed that the glass substrate covered with black dye has two prominent absorption bands at around 451 nm and 624 nm, accompanied by a less pronounced peak at around 571 nm. The overall absorption of the substrate is approximately 0.8, which may be attributed to its thickness of approximately 301 µm. The observed increased absorption of the black dye at these specific wavelengths can be attributed to the resonance of the canonical structures present in the ring opening form, as depicted in Scheme 1 [14].
When the black dye was heated to 40 oC the absorption bands were no longer observed (Figure 2B), and the overall absorbance decreased from 0.80 to 0.61. Based on this finding, it can be inferred that the black dye is composed of CVL leuco dye, and the ring opening and shutting mechanism takes place at temperatures of 20 oC and 40 oC each. Figure 2A illustrates the absorption spectrum of the three-component system, consisting of blue dye and binder, at room temperature. The spectrum spans from 501 nm to 651 nm, with a peak at around 625 nm and a shoulder at 551 nm. This absorption band is specific to the ring opening structure of CVL. The blue dye's absorbance (0.80-0.95) at room temperature (Figure 2A) decreases by a minimum of 50% when the sample is heated to 40 oC (Figure 2B) due to the closure of the ring. The absorbance of the SiO2-encapsulated blue dye remains constant between ambient temperature and 40 oC, owing to the minimal concentration of the dye particles. The observed phenomenon can be attributed to the dye's presence within the material with a significantly low refractive index (ɳ), specifically SiO2 with an index of 1.460. In a similar vein, the UV-Vis absorbance spectrum for the black or blue dye coated glass substrates with numerous TiO2 layers exhibits a consistent pattern within the wavelength range of 400 – 800 nm, with values ranging from 0.2 to 0.6. Nevertheless, the absorptions of these substances are comparatively elevated when they are enclosed within particles with a relatively high refractive (ɳ= ~2.0, TiO2).
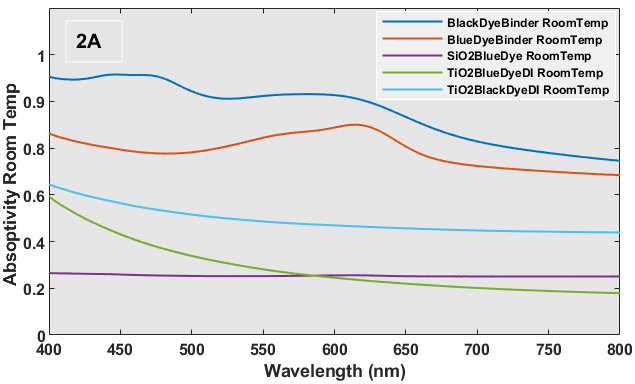
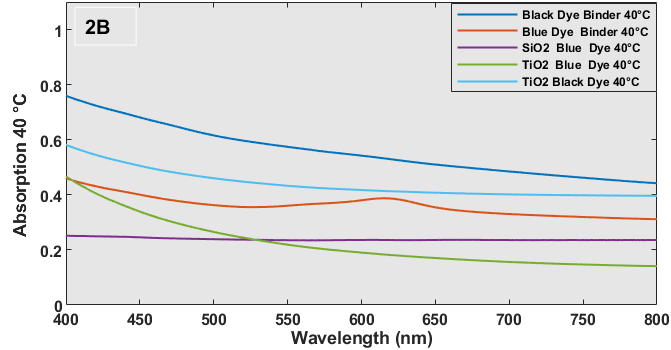
Figure 2 (C and D) presents spectra that depict the relationship between % transmission and wavelength. In general, the transmission curves of the plain dye/binder samples exhibit a rise in the percentage of transmission from 10-20% to 20-50% due to the distinct color change properties, transitioning from black or blue to white. This shift in color is highly reflective and advantageous for construction purposes. The alteration in hue would result in dispersion within the visible spectrum, leading to a modification in the refractive index. In this scenario, a rise in refractive index would indicate a modification in reflectance characteristics. The optical transmission of individual metal oxide encapsulated thermochromic dyes exhibits minimal or no alteration when subjected to a temperature of 40 oC, in contrast to samples maintained at room temperature. However, a modification in transmission characteristics occurs when encapsulation is achieved using several oxide systems. Typically, silica-based encapsulation exhibits excellent transmission due to its extremely low refractive index of 1.46, but titania-based encapsulation has reduced transmission due to its refractive index being close to 2.0. The reciprocal effect will be observed in the case of reflectance spectra.
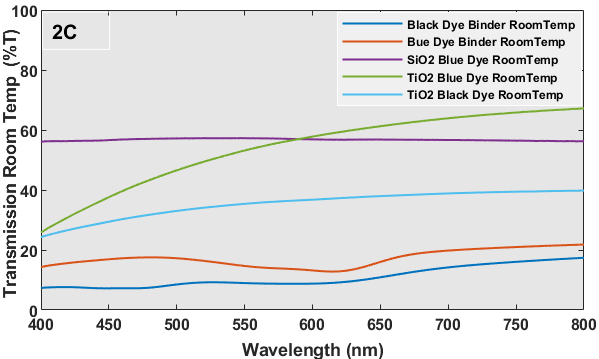
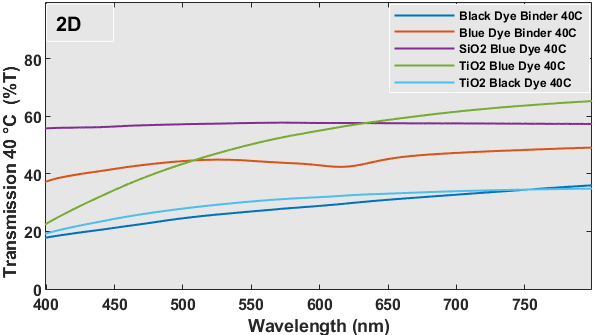
The UV-Vis reflectance spectra of each sample at temps of 20 oC (room temperature) and 40 oC for wavelengths spanning from 400-800 nm are depicted in Figure 2 (E and F). Despite being brought to the UV-Vis equipment at a temperature of approximately 40 oC, the samples experience inherent heat loss as a result of the extensive scanning procedure required to cover the whole wavelength range. Hence, Figure 2 (E and F) exhibited certain disparities in reflectance. On the glass substrate, the simple dye with a binder coating exhibits a greater reflection as a result of its chromogenic properties. This means that the dye changes color from black or blue to white, leading to dispersion and a relatively high reflectance. No significant alteration in reflectance was seen for the metal oxide capsule or multilayered coatings of these dyes. The blue dye exhibits a highly conspicuous band at approximately 626 nm. Figure 3 shows the heat-treated samples at 40 oC and room temperature in each sample were tested for this experiment.
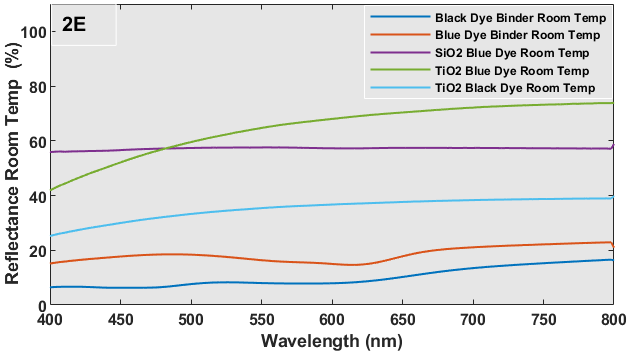
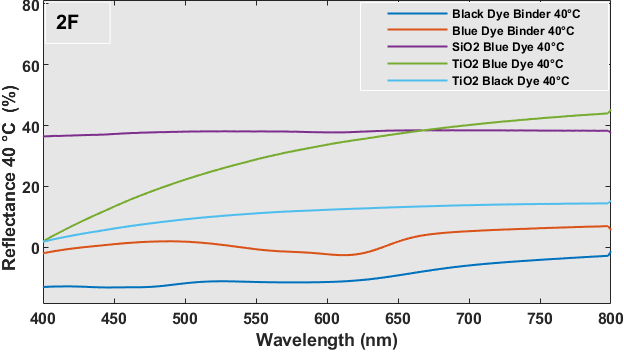
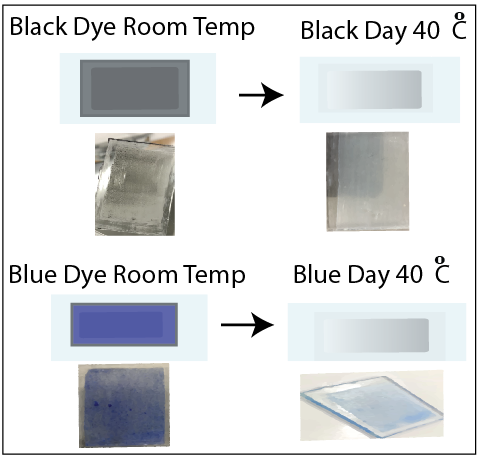
5. Conclusion
The fabrication process involved the creation of six distinct thin films, as outlined in Table 1. The UV-Vis spectral properties of these films were then examined, specifically focusing on absorbance, percent transmission, and percent reflection. The incident wavelengths used for this study ranged from 200 to 800 nm, and the films were subjected to both room temperature and heat treatment at 40 oC. This paper presents major spectra obtained within the visible region, specifically within the wavelength range of 400 nm to 800 nm. Distinct patterns are observed in the absorption spectra of the ordinary black and blue dyes. However, these features vanish when the dyes are heated to 40 oC due to molecular chemical changes and the resulting color change. The quantitative analysis and reporting of the color change behavior of these systems have been conducted for the samples at two distinct temperatures utilizing CIE LAB. The primary determinant of chromogenic behavior is the brightness index. The color shift behavior from black to white or blue to white is characterized by a positive value of the color difference, dL*, and the energy difference, dE*. The thin films on the glass substrate exhibit a surface morphology characterized by a consistent covering of well-defined spherical particles, similar to the commercial black dye that was pre-encapsulated with polymer layers. The energy-dispersive X-ray spectroscopy (EDS) profiles clearly indicate the presence of carbon, silicon, and oxygen, which can beattributed to the dye particles and the sodium silicate binder. The reversible thermochromic action of the blue dye and SiO2 contained blue dye is confirmed by the differential scanning calorimetric profiles. The enthalpic values obtained from both the heating and cooling cycles (ranging from 10-14 mWoC) indicate the thermal storage properties of these materials, suggesting their potential application in building envelopes.
Acknowledgements
The authors are thankful to the Deanship of Graduate Studies and Scientific Research at University of Bisha for supporting this work through the Fast-Track Research Support Program.
References
[1] A. Dimoudi, S. Zoras, A. Kantzioura, X. Stogiannou, P. Kosmopoulos, and C. Pallas, "Use of cool materials and other bioclimatic interventions in outdoor places in order to mitigate the urban heat island in a medium size city in Greece," Sustainable Cities and Society, vol. 13, pp. 89-96, 2014.
View Article
[2] A. J. Arnfield, "Two decades of urban climate research: a review of turbulence, exchanges of energy and water, and the urban heat island," International Journal of Climatology: a Journal of the Royal Meteorological Society, vol. 23, no. 1, pp. 1-26, 2003.
View Article
[3] M. Santamouris, "Using cool pavements as a mitigation strategy to fight urban heat island-A review of the actual developments," Renewable and Sustainable Energy Reviews, vol. 26, pp. 224-240, 2013.
View Article
[4] N. L. Alchapar, E. N. Correa, and M. A. Cantón, "Classification of building materials used in the urban envelopes according to their capacity for mitigation of the urban heat island in semiarid zones," Energy and Buildings, vol. 69, pp. 22-32, 2014.
View Article
[5] R. Baetens, B. P. Jelle, and A. Gustavsen, "Properties, requirements and possibilities of smart windows for dynamic daylight and solar energy control in buildings: A state-of-the-art review," Solar energy materials and solar cells, vol. 94, no. 2, pp. 87-105, 2010.
View Article
[6] M. Kolokotroni, B. Gowreesunker, and R. Giridharan, "Cool roof technology in London: An experimental and modelling study," Energy and Buildings, vol. 67, pp. 658-667, 2013.
View Article
[7] Revel, G. M., Martarelli, M., Emiliani, M., Celotti, L., Nadalini, R., De Ferrari, A., ... & Beckers, E. (2014). Cool products for building envelope-Part II: Experimental and numerical evaluation of thermal performances. Solar energy, 105, 780-791.
View Article
[8] P. A. Leitl, J. Smoker, M. L. Garcia de Albeniz and A. Flanschger ""Numerical and experimental investigation of different Riblet layouts on a Stratos 716 X business jet," AIAA SCITECH 2023 Forum, 2023
View Article
[8] A. Seeboth, R. Ruhmann, and O. Mühling, "Thermotropic and thermochromic polymer based materials for adaptive solar control," Materials, vol. 3, no. 12, pp. 5143-5168, 2010.
View Article
[9] Y. Ma, B. Zhu, and K. Wu, "Preparation of reversible thermochromic building coatings and their properties," Journal of Coatings Technology, vol. 72, no. 911, pp. 67-71, 2000.
View Article
[10] Hakami, A., Indrakar, S., Krishnegowda, A., Huang, M. Y., Sahebkar, K., Biswas, P. K., ... & Srinivasan, S. (2021). Microencapsulation of thermochromic material by silicon oxide nanoparticles. Key engineering materials, 878, 41-48.
View Article
[11] A. Hakami, S. S. Srinivasan, P. K. Biswas, A. Krishnegowda, S. L. Wallen, and E. K. Stefanakos, "Review on thermochromic materials: development, characterization, and applications," Journal of Coatings Technology and Research, vol. 19, no. 2, pp. 377-402, 2022.
View Article
[12] O. Panák, M. Držková, and M. Kaplanová, "Insight into the evaluation of colour changes of leuco dye based thermochromic systems as a function of temperature," Dyes and Pigments, vol. 120, pp. 279-287, 2015.
View Article
[13] M. I. Khan, K. Azizli, S. Sufian, and Z. Man, "Sodium silicate-free geopolymers as coating materials: Effects of Na/Al and water/solid ratios on adhesion strength," Ceramics International, vol. 41, no. 2, pp. 2794-2805, 2015.
View Article
[14] K. Takaoka, S. Maeda, H. Miura, K. Endo, and D. P. Chong, "Theoretical Valence XPS and UV-Visible Absorption Spectra of Four Leuco Dyes Using MO Calculations," Bulletin of the Chemical Society of Japan, vol. 71, no. 4, pp. 807-816, 1998.
View Article
[1] A. Dimoudi, S. Zoras, A. Kantzioura, X. Stogiannou, P. Kosmopoulos, and C. Pallas, "Use of cool materials and other bioclimatic interventions in outdoor places in order to mitigate the urban heat island in a medium size city in Greece," Sustainable Cities and Society, vol. 13, pp. 89-96, 2014. View Article
[2] A. J. Arnfield, "Two decades of urban climate research: a review of turbulence, exchanges of energy and water, and the urban heat island," International Journal of Climatology: a Journal of the Royal Meteorological Society, vol. 23, no. 1, pp. 1-26, 2003. View Article
[3] M. Santamouris, "Using cool pavements as a mitigation strategy to fight urban heat island-A review of the actual developments," Renewable and Sustainable Energy Reviews, vol. 26, pp. 224-240, 2013. View Article
[4] N. L. Alchapar, E. N. Correa, and M. A. Cantón, "Classification of building materials used in the urban envelopes according to their capacity for mitigation of the urban heat island in semiarid zones," Energy and Buildings, vol. 69, pp. 22-32, 2014. View Article
[5] R. Baetens, B. P. Jelle, and A. Gustavsen, "Properties, requirements and possibilities of smart windows for dynamic daylight and solar energy control in buildings: A state-of-the-art review," Solar energy materials and solar cells, vol. 94, no. 2, pp. 87-105, 2010. View Article
[6] M. Kolokotroni, B. Gowreesunker, and R. Giridharan, "Cool roof technology in London: An experimental and modelling study," Energy and Buildings, vol. 67, pp. 658-667, 2013. View Article
[7] Revel, G. M., Martarelli, M., Emiliani, M., Celotti, L., Nadalini, R., De Ferrari, A., ... & Beckers, E. (2014). Cool products for building envelope-Part II: Experimental and numerical evaluation of thermal performances. Solar energy, 105, 780-791. View Article
[8] P. A. Leitl, J. Smoker, M. L. Garcia de Albeniz and A. Flanschger ""Numerical and experimental investigation of different Riblet layouts on a Stratos 716 X business jet," AIAA SCITECH 2023 Forum, 2023 View Article
[8] A. Seeboth, R. Ruhmann, and O. Mühling, "Thermotropic and thermochromic polymer based materials for adaptive solar control," Materials, vol. 3, no. 12, pp. 5143-5168, 2010. View Article
[9] Y. Ma, B. Zhu, and K. Wu, "Preparation of reversible thermochromic building coatings and their properties," Journal of Coatings Technology, vol. 72, no. 911, pp. 67-71, 2000. View Article
[10] Hakami, A., Indrakar, S., Krishnegowda, A., Huang, M. Y., Sahebkar, K., Biswas, P. K., ... & Srinivasan, S. (2021). Microencapsulation of thermochromic material by silicon oxide nanoparticles. Key engineering materials, 878, 41-48. View Article
[11] A. Hakami, S. S. Srinivasan, P. K. Biswas, A. Krishnegowda, S. L. Wallen, and E. K. Stefanakos, "Review on thermochromic materials: development, characterization, and applications," Journal of Coatings Technology and Research, vol. 19, no. 2, pp. 377-402, 2022. View Article
[12] O. Panák, M. Držková, and M. Kaplanová, "Insight into the evaluation of colour changes of leuco dye based thermochromic systems as a function of temperature," Dyes and Pigments, vol. 120, pp. 279-287, 2015. View Article
[13] M. I. Khan, K. Azizli, S. Sufian, and Z. Man, "Sodium silicate-free geopolymers as coating materials: Effects of Na/Al and water/solid ratios on adhesion strength," Ceramics International, vol. 41, no. 2, pp. 2794-2805, 2015. View Article
[14] K. Takaoka, S. Maeda, H. Miura, K. Endo, and D. P. Chong, "Theoretical Valence XPS and UV-Visible Absorption Spectra of Four Leuco Dyes Using MO Calculations," Bulletin of the Chemical Society of Japan, vol. 71, no. 4, pp. 807-816, 1998. View Article