Volume 10 - Year 2023 - Pages 01-09
DOI: 10.11159/jffhmt.2023.001
Phase Change Materials for Better Thermal Performance of Central Processing Units (CPUs)
S. Aal Khlaifin1, A. Al-Janabi1, N. Al-Rawahi1, N. Al-Azri1
1Sultan Qaboos University, College of Engineering, Department of Mechanical and Industrial Engineering,
P.O. Box: 33, P.C. 123 Alkhoud, Muscat, Sultanate of Oman, *corresponding author: ab.aljanabi@squ.edu.om
Abstract - Nowadays, the small size of electronic components causes an increase in the heat flux that is dissipated at their surfaces, consequently, efficient cooling systems are required to improve reliability, prevent premature failure as well as keep components within permissible operating temperature limits. Most electronic devices use forced air-cooling which nowadays is somehow not adequate or even enough to keep the system cool. This study is aimed at investigating a passive cooling technique using the phase change material (PCM) type (RT35HC) to absorb the generated heat at the CPU of a personal computer and keep its surface temperature within an acceptable range. To do so, a set of 20 experiments were conducted where four different amounts of the PCM (20, 40, 60, and 80g) were subjected to five levels of dissipated heat (10, 20, 30, 40, and 50W). The results showed using RT35HC regardless of its amount enhanced the thermal performance of the CPU and provided a longer operating period while keeping the CPU surface temperate constant for low levels of dissipated heat (10-20W). On the other hand, increasing the PCM amount could help in providing a better thermal performance of the CPU that dissipated a higher amount of heat (30-50W) compared to thermal performance without using the PCM. The optimum amount of the PCM that can be used for passive cooling of the CPU was found to be 60g.
Keywords: Phase Change Materials, CPU, Passive Cooling, Thermal Management.
© Copyright 2023 Authors - This is an Open Access article published under the Creative Commons Attribution License terms Creative Commons Attribution License terms. Unrestricted use, distribution, and reproduction in any medium are permitted, provided the original work is properly cited.
Date Received: 2023-12-14
Date Revised: 2023-01-23
Date Accepted: 2023-01-30
Date Published: 2023-02-08
1. Introduction
The passage of electric current through a resistance of the electronic components leads to generating heat as a side effect of the process. The rapid development in electronic components and their usage requires further miniaturization of their size which could increase the complexity of effectively dissipating the generated heat [1] The generated heat in the electronic components is considered a disadvantageous feature that could lead to increasing the working temperature and affect the reliability and performance of the device in many ways like thermal shock, thermal de-bonding, and thermal fracture mechanical stresses [2]. The failure rate of electronic equipment increases exponentially with temperature [3]. Yeh [4] concluded that 55% of failures in electronics were associated with increases the temperature and the reliability of a silicon chip decreased by 10% for every 2°C increase in temperature [5]. Because higher-performance complex electronic devices such as smartphones and laptop computers must be light in weight and small in size, this problem has become much more challenging [6]. The components that can malfunction or suffer from permanent failure if overheated include CPUs (Central Processing Units), chipsets, graphics cards, hard disk drives, etc. So, to control the temperature and prevent electronic components from overheating, the maximum permissible temperature is set to be within the range of 85oC to 120˚C [7]. Therefore, thermal management has become the most significant aspect of the design and operation of electronic components. The market of thermal management technologies was valued at $14.5 billion in 2021 and should grow to $20.9 billion by 2026 with a compound annual growth rate (CAGR) of 7.6% for the period of 2021-2026 [8]. Most electronic devices use forced air-cooling to dissipate the generated heat, but for some applications that generate excess amounts of heat, air-cooling is somehow not adequate or even enough to keep the system cool [9]. So, liquid cooling might be a better choice to increase the efficiency of the cooling system. This, in fact, belongs to the higher convection heat transfer coefficients associated with liquids that could develop a better heat transfer rate in comparison to that of air-cooling systems. However, this system has a disadvantage associated with the direct contact of the water, in case of leakage, with electronic components which could lead for example to damage to the CPU and causes corrosion in its components [10]. Accordingly, thermal management is turning into necessary and progressively important to the electronics field and has become a challenging aspect of electronic device design. The task of maintaining an acceptable junction temperature by dissipating heat from the computer circuit chips is a significant challenge for thermal engineers. In addition, the huge conventional air-cooled systems are no longer applicable since electronic device packages have become smaller. As a result, new ways of keeping electronic packages within the functioning temperature range are being developed.
The use of Phase Change Materials (PCMs) has gotten a lot of interest, because of their high latent heat and low volume change during phase transition, solid-liquid phase change materials are more popular. Organic PCMs are very valuable because of their compatibility with other materials and safety [11]. In general, during a solid-to-liquid phase transition, PCM absorbs thermal energy and stores it. So, this allows the temperature of the electronics to be maintained near the PCM's melting point. PCMs are found to have considerable advantages and disadvantages as compared to other available options for thermal management applications [12]. The use of PCMs for transient thermal management has the benefit of keeping a constant system temperature throughout the melting process, regardless of the heat flux applied. On the other hand, the disadvantages of PCMs are low thermal conductivity and super-cooling, [13]. Gaikwad and More [14] did an experimental study to compare the cooling process using PCM in microchannel heat sinks and water-cooling systems to cool down computer processors. It has been found that microchannel heat sinks with PCM as coolant is more efficient compared to conventional cooling as well as water cooling system. However, this cooling technique cannot be used in transient operations as the temperature of the CPU may go beyond the limitations of Thermal Design Power (TDP). The TDP is the maximum power for which the system should be designed. Taremi, [11] found that combining PCMs and heat sinks is one of the best cooling system solutions. Phase change materials, as previously stated, can absorb a significant amount of overload heat without raising the temperature during peak chip activity. The operational temperature range of a certain electronic package can be modified by selecting the right phase change material. However, the research area of cooling the electronics components, especially the CPUs using the phase change material (PCM) is still not saturated and required more effort to quantify the superior performance of the PCM and enrich this area with available scientific experimental data. Accordingly, the present experimental study is an attempt in this direction to develop a practical and feasible solution to improve the thermal performance of the existing cooling system using PCM type RT35HC as a passive cooling technique to enhance the thermal management of the CPU of the personal computer working under the steady operating condition where the power supplied to the CPU remains constant.
2. Experimental Setup and Procedure
The experimental setup as shown in Figure 1 consists of the CPU unit, the measurement equipment, and the PCM. From a practical point of view, using a real CPU might be not the right choice to conduct the experiments where many parameters will be investigated. Accordingly, a simulated CPU was designed and constructed using a copper plate of size 45 cm x 45 cm x 5 mm which is closer to the real size of the most common CPUs. A power supply was used to serve as a source of electrical power to simulate the dissipated heat from the CPU. The electric power supply was linked to a wire resistance made of Nichrome with a resistance of 12.2 Ohm. The device has a maximum current of 3 amps and a maximum voltage of 30 volts.
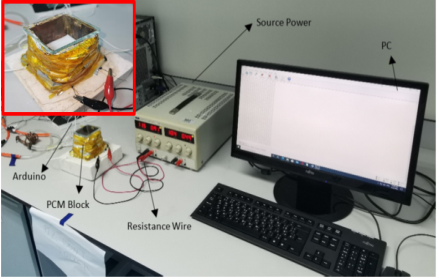
Phase change material type RT35HC was used to perform the passive cooling technique that is required to maintain the CPU temperature within safe operating conditions. Due to its high heat storage capacity (240 kJ/kg), it has the ability to absorb a significant amount of thermal energy during the melting process while keeping the CPU surface temperature at a constant value. The physical properties of the utilized PCM type RT35HC are listed in Table 1.
Table. 1 Physical properties of the PCM type RT35HC
Properties |
Value |
unit |
Melting temperature |
34-36 |
oC |
Heat storage capacity |
240 |
kJ/kg |
Heat conductivity (both phases) |
0.2 |
W/m. K |
Volume expansion |
12.5 |
% |
The CPU surface temperature, the temperature of the PCM, and the ambient temperature were measured using NTC100K thermistors with a resolution of ± 0.01oC. Arduino model ATmega328P was used to record the readings of the thermistors and the power supplied during the experiments. To better understating the thermal performance of the PCM, four different quantities of RT35HC were used as a heat absorber material, to absorb the heat from a simulated CPU. They were prepared using a digital scale, i.e., 20g, 40g, 60g, and 80g. In addition, the CPU was subjected to four different levels of dissipated heat, e.g., 10W, 20W, 30W, 40W, and 50W.
Each specific amount of the PCM was subjected to the four levels of dissipated heat for a time period of 90 minutes. For better quality of the quantitative data, the temperatures were measured every 30 seconds. Visual observation was done in order to track the melting process of the PCM and have a clear picture of its thermal behavior during the experiments. At the beginning of each experiment, a specific amount of the PCM was prepared and inserted in the PCM block and covered by a sheet of glass that provides the possibility for visual observation.
After that, the power source and the PC were both switched on to start the experiment. The current and the voltage of the heat source were both set up to provide a specific amount of dissipated heat. The Arduino program was started to record the thermocouples reading with a time interval of 30 seconds. This process was conducted till reached the steady state condition for the CPU surface temperature. Later on, the Arduino program was stopped, and the recordings were saved by using the CoolTerm – Shortcut application. The power source and the PC were both switched off. The PCM was left for a certain time until it solidified again.
3. Results Analysis and Discussion
This section is devoted to analyzing the experimental data in terms of the CPU surface temperature profile and the PCM temperature profile in order to come up with behavior explanations, parameter effects, findings, and future suggestions.
3.1 CPU Thermal Performance without Cooling
The surface temperature of the CPU was monitored while various amounts of power were supplied (dissipated heat) without cooling (which means without using the PCM). Figure 2 shows the temperature profile of the CPU as a function of time at a different level of dissipated heat. By applying the fundamental concept of the first law of thermodynamics for a closed as illustrated in Figure 2, the amount of dissipated heat is in fact the amount of the supplied power to the CPU as expressed in equation (1.1).
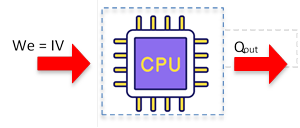

Where is the supplied power to the CPU in terms of the current (I) and the voltage (V),
is the dissipated heat from the CPU, ∆KE is the change in the kinetic energy which is in the CPU equals zero, the same as for the change in the potential energy ∆PE. Accordingly, equation (1.1) rewritten to be as:

Figure 3 shows the temperature profile of the CPU as a function of time at a different level of dissipated heat. The CPU temperature profile for each amount of dissipated heat started with a sharp increase. The temperature started rising in relatively a linear slope from its initial condition at approximately 24oC (room temperature). After a specific period of time, the CPU surface temperature reached asymptotic behavior where the temperature remained almost constant. The CPU without the existence of any cooling system was only subjected to the natural convection mode as a result of natural air movement generated by bouncy force associated with density variation with temperature. Despite the fact that the rate of heat transfer by natural convection is increased by increasing the surface temperature as depicted in equations (1.3) [15] the natural convention was not sufficient to remove the generated heat on the CPU and consequently the CPU surface temperature reached to extremely high range. This finding was in line with that achieved by [16].
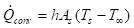
The slope of the sharp increase and the level of asymptotic temperature increase with increasing the dissipated heat value.
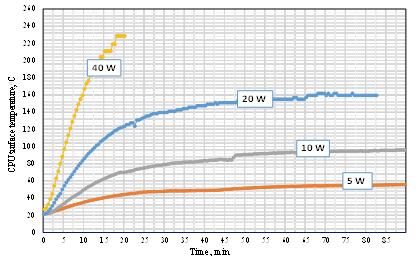
Table 2 shows some quantitative data extracted from Figure 3. Without using a cooling system, the CPU surface temperature reached values above 100oC (The most common thermal design temperature of the CPUs), especially when the CPU is subjected to dissipated heat above 20W.
Table 2: CPU temperature profile characteristics without a cooling system
Parameters |
Dissipated heat (W) |
|||
05 |
10 |
20 |
40 |
|
Tmax, (oC) |
55.7 |
95.9 |
161.8 |
228.67 |
Constant temperature period, (min) |
30-50 |
30-40 |
25-40 |
-- |
The linear part slope, (℃/min) |
3.54 |
5.03 |
8.63 |
16.71 |
Ts at the end of the linear part, (oC) |
36.62 |
53.0 |
91.79 |
135.64 |
For safety conditions related to the simulated CPU, the experiment under 40W was turned off at 20 minutes as the surface temperature reached approximately 230oC. Based on these observations, without a cooling system, the CPU surface temperature reached to level which is extremely harmful to any sort of electronic device, not just the CPU, because increasing the temperature plays a decisive role in reducing reliability and resulting in poor performance of the electronic components [17]. In the case of the CPU, this might result in slower clocking speeds and, eventually, the system burning, potentially resulting in fires [18]. A suitable cooling system is required to avoid these incidents.
3.2 CPU Thermal Performance under Passive Cooling
In this section, the thermal performance of the CPU subjected to a passive cooling system using the PCM was discussed. The temperature profile of the CPU and the PCM was discussed and explained. Each quantity of PCM (i.e., 20g, 40g, 60g, 80g) was tested five times at different heat sources (i.e., 10W, 20W, 30W, 40W, 50W) in a total of 20 experiments. For each experiment, the readings of each thermocouple were gathered and explained as follows:
3.2 CPU Thermal Performance under Passive Cooling
Figure 4 shows the profile of the CPU surface temperature subjected to variable levels of the dissipated heat using four different amounts of PCM. The temperature profile using PCM-TR35MC has a different trend than that without cooling. It started with an almost linear increase in temperature for a short period of time followed by a period where the surface temperature increased gradually till reaching a constant value for a specific period of time.
After that the surface temperature started again to increase till reaching the asymptotic profile in some cases. It is worth mentioning that the period of constant surface temperature is referred to the melting region of the PCM where the dissipated heat was observed to change the PCM from the solid phase to the liquid phase. The duration of this period was strongly affected by the amount of dissipated heat and the amount of the utilized PCM as shown in Figure 4. In case the CPU is subjected to a lower level of power (e.g., 10-20W), using PCMs as a passive cooling system is a suitable solution to prevent the TCPU to become above the design value. The CPU can work for a long period of time while the TCPU is below 100oC.
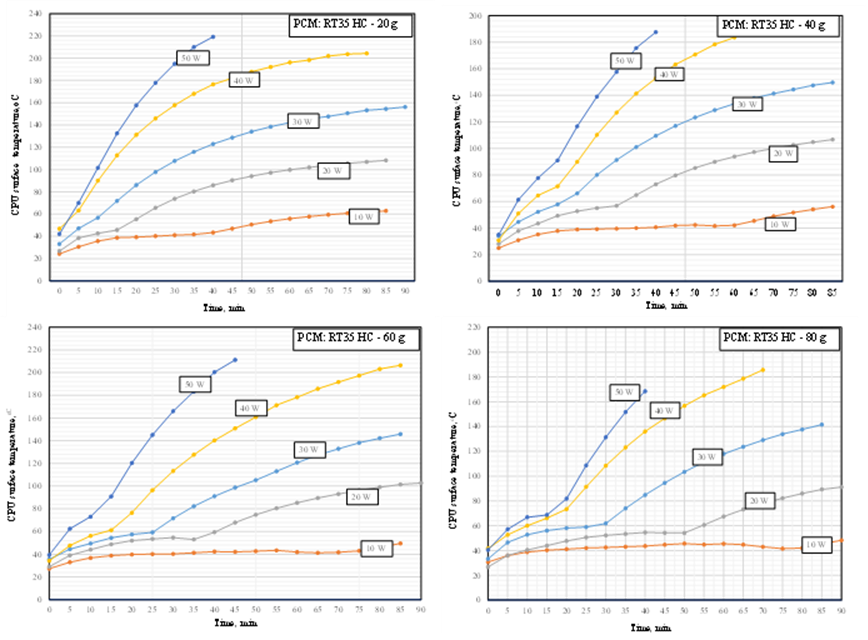
The amount of the dissipated heat was absorbed by the PCM in terms of sensible heat and latent heat. It has been found that at a very low level of dissipated heat (e.g., 10-20W) longer time was required for the melting region when a larger amount of PCM was used. This indicated that the dissipated heat was not enough to melt the whole amount of the PCM. For 80 g, the PCM required 70 minutes to complete the melting process, and the maximum temperature after 90 minutes was 48oC. So, using the PCM as a passive cooling system seems a suitable solution to prevent the CPU temperature to become above the thermal design point (TDP). This guarantees that the system operates according to the specified specifications even when subjected to the maximum theoretical demand [19]. In the case of considering the maximum working temperature of the CPU to be 100oC, the results presented in Figure 4 showed that the PCM of 60g and 80g provide better cooling in terms of a longer period of constant surface temperature that was below the maximum design limits of the CPU surface temperature.
3.2.2 CPU Surface Temperature with Cooling vs. without Cooling
The surface temperature profile of the CPU at 20W and under passive cooling using 60g of PCM-RT35HC was compared with that without cooling as shown in Figure 5. This is done, to demonstrate how the modified cooling system affects the CPU surface temperature. Figure 5 shows that the CPU surface temperature profile is nearly identical for the first three minutes whether the system is being cooled or not. However, at around 3.5 minutes, regardless of the quantity of PCM utilized, a new trend begins to appear.
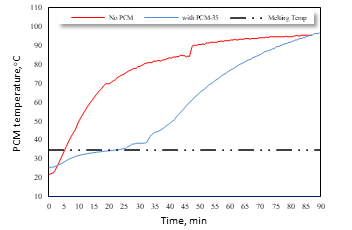
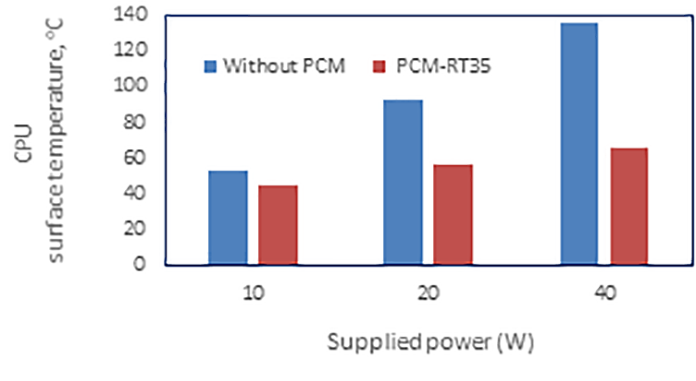
The CPU surface has been tested with and without PCM filled in the CPU block. It has been found that the CPU surface temperature (without PCM) increased rapidly and the temperature during steady state reached 5.97oC, 161.88◦C, and 228.67oC for power ratings of 10W, 20W, and 40W, respectively. This rapid increase in temperature in electronic devices is not desired and affects their performance. Figure 6 is extracted from Figure 5.4 and shows a comparison between the CPU surface temperature during the thermal regulation period for a CPU filled with PCM (RT35) and without PCM. The CPU surface temperature is markedly decreased compared to no PCM by 45°C, 56°C, and 66°C for 10, 20, and 40W power ratings, respectively.
The temperature drops (achieved using PCM) increase as the power rating increases. The results also show that the duration of thermal regulation is inversely proportional to the supplied power for the same amount of PCM [20].
3.2.3 PCM temperature profile (Tpcm)
The thermal performance data of the PCM at 20g, 40g, 60g, and 80g are shown in Table 3.
Table 3: PCM-RT35HC characteristics
Thermal performance data of PCM-RT35HC as passive cooling |
|||||
Mass (g) |
Dissipated heat (W) |
Solid sensible zone |
Latent heat zone |
Liquid sensible zone |
Tmax (oC) |
20 |
10 |
(21 - 34oC) |
(34 - 55oC) |
(55 - 60oC) |
60 |
20 |
(21 - 35oC) |
(35 - 80oC) |
(80 - 100oC) |
100 |
|
30 |
(23 - 34oC) |
(34 - 67oC) |
(67 - 145C) |
145 |
|
40 |
(26 - 35oC) |
(35 - 69oC) |
(69 - 193oC) |
193 |
|
50 |
(25 - 33oC) |
(33 - 68oC) |
(68 - 198oC) |
198 |
|
40 |
10 |
(22 - 34oC) |
(34 - 43oC) |
(43 - 53oC) |
53 |
20 |
(22 - 32oC) |
(32 - 52oC) |
(52 - 99oC) |
99 |
|
30 |
(24 - 35oC) |
(24 - 68oC) |
(68 - 142oC) |
142 |
|
40 |
(22 - 29oC) |
(29 - 69oC) |
(69 - 173oC) |
173 |
|
50 |
(22 - 32oC) |
(32 - 98oC) |
(98 - 183oC) |
183 |
|
60 |
10 |
(23 - 35oC) |
(23 - 39oC) |
(39 - 46oC) |
46 |
20 |
(25 - 34oC) |
(34 - 49oC) |
(49 - 96oC) |
96 |
|
30 |
(26 - 35oC) |
(35 - 54oC) |
(54 - 138oC) |
138 |
|
40 |
(23 - 30oC) |
(23 - 58oC) |
(58 - 170oC) |
170 |
|
50 |
(22 - 30oC) |
(22 - 102oC) |
(102- 172oC) |
172 |
|
80 |
10 |
(24 - 33oC) |
(33 - 40oC) |
(40 - 44oC) |
44 |
20 |
(22 - 32oC) |
(32 - 52oC) |
(52 - 84oC) |
84 |
|
30 |
(22 - 33oC) |
(33 - 56oC) |
(56 - 133oC) |
133 |
|
40 |
(24 - 35oC) |
(35 - 37oC) |
(37 - 164oC) |
164 |
|
50 |
(24 - 34oC) |
(34 - 74oC) |
(74 - 167oC) |
167 |
No matter how much PCM is used, the PCM temperature increases as the dissipated heat are raised. Additionally, for each particular quantity of dissipated heat, the PCM has a better temperature profile as the PCM amount increases. In conclusion, the PCM temperature profile has a reverse relationship to the PCM quantity but a proportional relationship to the dissipated heat. The PCM temperature profiles for 10W and 20W were less time-sensitive to the dissipated heat than 40W and 50W at any PCM quantity. For instance, the temperature profile at 80g PCM was almost linear at 10W of dissipated heat, however, it showed different behavior at 50W. At 10W, the PCM was still in the sensible heat region and did not melt. But at 50W, the PCM melted and moved into the latent heat region. It is evident that the PCM temperature remained constant for a while before once more entering the range of sensible heat for liquid. PCM reached the latent heat region at different periods depending on its quantity and the level of the dissipated heat. The maximum temperature that the CPU could reach increased as the level of dissipated heat or the PCM amount increased. However, the increase in the temperature was more sensitive to the changes in heat that is dissipated from the CPU than to the changes in the amount of PCM. For instance, if the PCM amount changes from 20g to 80g while the dissipated heat remains constant at 10W, the temperature decreases from 60°C to 44°C, whereas it increases to 211°C when the supplied heat is changed from 20W to 50W while the PCM amount remains constant at 20g. The PCM can reach a maximum temperature of 198°C at 20g and 50W, and a minimum temperature is 44°C at 80g and 10W.
Figure 7 shows a comparison between the real and the theoretical behaviors of the PCM temperature profile for 80g at 50W of the dissipated heat.
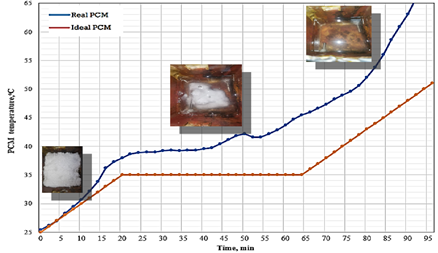
The real temperature profile was closer to that of the theoretical one with some deviations. The PCM was firstly subjected to an increase in its temperature till reaching the melting temperature of 34-35oC. This region is called the solid sensible region which required 15 minutes to reach this point. After that between 15 to 40 minutes, the PCM started to melt, which corresponds to the latent heat region. Until the entire solid was converted to liquid in the next 25 minutes, the temperature profile remained essentially constant. The PCM entered the liquid sensible heat region after 15 minutes after leaving the melting region. In this region, the PCM temperature increased rapidly and the PCM melted completely.
4. Conclusions
Due to the significant development in electronic devices to be in smaller sizes to match the required industrial, the generated heat flux from them increases rapidly. Consequently, an efficient cooling system is required to maintain their performance to be within the design conditions. A passive cooling technique using the PCM was investigated in this study with the aim of achieving better thermal performance of the CPU of personal computers. To do so, various scenarios were evaluated in order to look into how well the cooling system performed. Four amounts of PCM: 20g, 40g, 60g, and 80g of PCM were used to monitor the effects of the amount and select the best value for large-scale applications. These amounts were subjected to five levels of dissipated heat i.e., 10W, 20W, 30W, 40W, and 50W, that are simulating the heat flux produced by a real CPU. These values represent typical usage (20W and 30W) and heavy use (40W and 50W). The results demonstrated a considerable variation in CPU surface temperature when subjected to passive cooling compared to that without cooling. It has been found that using the PCM type RT35HC provide better thermal management (even with a low amount) for the CPUs that are dissipating lower level of heat while in process. The high heat capacity value of the PCM allowed the CPU to work probably without facing a significant increase in its surface temperature. However, once the dissipated heat level increases, the low amount of the PCM was no longer enough to absorb the generated heat from the CPU. However, increasing the amount of PCM could enhance the performance of the CPU. It has been found that 60-80g of PCM developed an optimum thermal performance for the CPU at a low level of the dissipated heat and an acceptable working performance at a high level of dissipated heat.
References
[1] F. Mustapha and H. Qarnia, E. Lakhal, "Thermal analysis of a phase change material-based heat sink for cooling protruding electronic chips," Journal of Thermal Science, vol. 18, pp. 268-275, 2009. View Article
[2] S. Ramalingam, and R. Velraj "Thermal Managements of Electronics: A Review of Literature," Thermal Science, vol. 12, no.2, pp. 5-26, 2008. View Article
[3] J. Watson and G. Castro, "High-Temperature Electronics Pose Design and Reliability Challenges," Analog Dialogue, pp. 46-04, 2012.
[4] L. T. Yeh, "Review of heat transfer technologies in electronic equipment," Journal of Electronic Packaging, Transactions of the ASME, vol. 117, pp. 333-339, 1995. View Article
[5] P. Wang and A. Bar-Cohen, "On-chip hot spot cooling using silicon thermoelectric microcoolers," Journal of Applied Physics, vol. 102, pp. 034503, 2007. View Article
[6] M. Faraji and H. Qarnia, "Passive Cooling of Protruding Electronic Components by Latent Heat of Fusion Storage," J. of Electronic Packing, vol. 131, no. 2, pp. 021011, 2009. View Article
[7] Intel, "intel® coretm i7-7700 cpu @ 3.6ghz," 2017. [Online]. Available: View Article
[8] The Market for Thermal Management Technologies Market analysis report, May 2022, BCC Publishing.
[9] A. B. Etemoglu, "A brief survey and economical analysis of air cooling for electronic equipment," International Communications in Heat and Mass Transfer, vol. 34, no 1, pp. 103-113, 2006. View Article
[10] S. Kumar, "Pap Thermal Management of RF and Digital Electronic Devices," American Standard Circuits, Inc., pp. 1-26, 2012.
[11] N. Taremi, "Optimization of Phase Change Material (PCM)-Based Heat Sink for Electronics Cooling Application," Master of Science Dissertation, University of California, 2017.
[12] R. J. Warzoha, R. M. Weigand, and A. S. Fleischer, "Temperature-dependent thermal properties of a paraffin phase change material embedded with herringbone style graphite nanofibers," Applied Energy, vol. 137, pp. 716-725, 2015. View Article
[13] I. Zarma, A. Abdel-Rahman, M. Ahmed, and S. Ookawara, "Investigation on Enhancement of Thermal Conductivity of Phase Change Material with Nanoparticles Addition for Thermal Energy Storage," 16th International Conference on Sustainable Energy Technologies- SET 2017, Bologna, IT.
[14] V. P. Gaikwad and S. P. More, "CPU Processor Cooling by using Microchannel Heat Sink with PCM as Coolant," International Journal of Engineering Research & Technology, vol. 6, no. 11, pp. 214-219, 2017.
[15] Y. Cengel, A. Ghajar. (2015). Heat and Mass Transfer: Fundamentals & Applications Third Edition, McGraw-Hill.
[16] A. Al-Janabi, H. Al-Busaidi, A. Alsawafi, A. Al-Yahmadi, and A. Almahruqi, "Enhancing the Thermal Performance of Electronic Components using Phase Change Materials," 2nd International Conference on Engineering and Applied Natural Sciences, October 15-18, 2022, Konya, Turkey.
[17] V. Lakshminarayanan and N. Sriraam, "The effect of temperature on the reliability of electronic components", Conference: 2014 IEEE, (CONECCT) View Article
[18] Peterson and Brown. (2012). Computer Networks a Systems Approach (5th ed), Morgan Kaufmann is an imprint of Elsevier.
[19] Intel, "8th and 9th Generation Intel® Core™ Processor Families Datasheet," 2020. [Online]. Available: View Article
[20] I. Al Siyabi, S. Khanna, T. Mallick and S. Sundaram "Multiple Phase Change Material (PCM) Configuration for PCM-Based Heat Sinks-An Experimental Study," Licensee MDPI, Basel, Switzerland, 2018. View Article