Volume 9 - Year 2022 - Pages 113-120
DOI: 10.11159/jffhmt.2022.015
Experimental Analysis of Initial Wettability Effect on the Performance of Hybrid Engineered Water-Assisted Polymer Flooding
Mariam Shakeel1, Peyman Pourafshary1, Muhammad Rehan Hashmet2
1Nazarbayev University, School of Mining & Geosciences
53 Kabanbay Batyr Avenue, Nur-Sultan, Kazakhstan 010000
Mariam.shakeel@nu.edu.kz; peyman.pourafshary@nu.edu.kz
2United Arab Emirates University, Chemical and Petroleum Department
Sheikh Khalifa Bin Zayed Street, Al Ain, Abu Dhabi
mrhashmet@uaeu.ac.ae
Abstract - Residual oil saturation (Sor) after waterflooding is high in carbonate reservoirs, due to the wettability state, making them a lucrative candidate for novel enhanced oil recovery (EOR) methods such as engineered water/polymer flooding (EWPF). Initial wettability for such reservoirs must be considered during the preliminary screening for an effective EWPF application. This experimental study investigates the carbonate initial wettability criteria to achieve a successful EWPF design. Contact angles are measured at 80 °C to evaluate the temperature effect on wettability shift by engineered water (EW). Coreflood tests are conducted under different initial wettability conditions to analyse EWPF performance in weak, moderate, and strong oil-wet mediums. Capillary numbers and a corresponding reduction in Sor are estimated to develop a screening parameter. EW prepared by 10 times diluted Caspian seawater spiked with optimized active ions has caused a 40-55° reduction in contact angle, making the rock more water-wet. Sor after waterflooding is 25% higher in a strong oil-wet carbonate sample in contrast with a weak oil-wet condition, implying such reservoirs are appropriate candidates for EWPF application. Ion-tuned water flooding has provided 8% of OOIC incremental recovery in strong oil-wet medium while it has given a negligible recovery in the weak oil-wet case. Despite having similar capillary numbers, EWPF has resulted in a 16% more reduction in Sor in the strong oil-wet system compared to the weak oil-wet system. This study presented a preliminary screening criterion for EW-based hybrid methods by considering the initial wettability of a reservoir. The results show that the hybrid EWPF method can reduce Sor more than that predicted by capillary desaturation curves. Hence, it has a higher capillary desaturation tendency and can be successfully applied to carbonate formations that are not water wet.
Keywords: Polymer flooding, wettability, enhanced oil recovery
© Copyright 2022 Authors - This is an Open Access article published under the Creative Commons Attribution License terms Creative Commons Attribution License terms. Unrestricted use, distribution, and reproduction in any medium are permitted, provided the original work is properly cited.
Date Received: 2022-09-19
Date Accepted: 2022-09-30
Date Published: 2022-10-13
1. Introduction
Hybrid engineered water/polymer flooding (EWPF) is an emerging technology that has a significant potential to enhance oil recovery, particularly from high salinity carbonate formations. Such reservoirs have different initial wettabilities and selection of the potential appropriate reservoir for the EWPF is challenging. Hybrid EWPF has been proven an efficient EOR technique by several experimental, analytical, and numerical modelling studies [1-9]. The EWPF method has shown an average 11% increase in the oil recovery from varying carbonate lithologies in different studies.
The increase in oil recovery by hybrid EWPF is due to the synergy between ion-tuned engineered water (EW) and polymer flooding (PF), leading to improved oil displacement. Owing to the salinity and composition gradient in the presence of engineered water, the wetting preference of the carbonate surface is altered from oil-wet to water-wet. As a result, the polar components in the crude oil such as negatively charged carboxylic materials are detached from the rock surface and are produced by the subsequent engineered water-polymer (EWP) flooding [10-12]. The governing mechanism of polymer flooding is to reduce the mobility of the displacing fluid, thereby lowering the mobility ratio and augmenting the volumetric sweep [13]. The oil production obtained by the hybrid EWPF increases beyond the recovery realized by the individual method. Furthermore, the thermal and salinity resistance of polymer increases in the presence of EW which leads to a lower polymer concentration requirement for the same target viscosity. This favourable interaction between EW and polymer can be exploited to extend PF application to carbonate formations with harsh conditions of temperature and salinity [7].
Literature shows that engineered water or chemically tuned low salinity water is capable of wettability alteration in carbonate rocks, however, it may not be always true depending upon the characteristics of the CBR system under examination. One of the important parameters to affect EWPF performance is the initial wettability of the reservoir. In some of the studies, little to negligible incremental recovery was obtained by low salinity water flooding due to the rock being water-wet or weak oil-wet [14-16]. Thus, it is necessary to develop a screening parameter in terms of the initial wettability of the reservoir for the application of the EWPF method. The initial wettability is an influencing factor for the capillary desaturation tendency of any EOR method. The ability of an enhanced oil recovery technique to cause a reduction in residual oil saturation by overcoming the capillary forces is termed the capillary desaturation tendency.
This paper presents a systematic study to evaluate the effect of initial wettability on the capillary desaturation tendency of the hybrid EWPF method in carbonate formations. The effect of EWPF on oil-brine-rock interactions at different wetting states is analysed by measuring surface properties and conducting oil displacement experiments. Application of EWPF affects capillary and viscous forces which result in lower residual oil saturation. Different studies have been conducted to analyse rock/fluids interaction and oil displacement at different temperatures and different initial wettability states i.e., weak oil-wet, intermediate-wet, and strong oil-wet. Injection of engineered water enhances the capillary number by altering rock surface properties. Switching to engineered water/polymer increases the capillary number by affecting viscous force by polymer and capillary force by EW.
2. Methodology
To study the effect of initial wettability on the performance of the hybrid EWPF method, a stepwise methodology is followed. The first step included the preparation of the materials to be used in the study and the determination of the required fluid and rock properties. In the second step, contact angles were measured to study the effect of aging time, temperature, and polymer on EW wettability alteration capability. In the third step, the core samples were aged at different times to achieve different initial wettability states. Finally, the coreflood tests were performed to quantify the incremental oil recovery as a function of initial wettability. This section presents the materials consumed and the procedures followed for the experiments.
2. 1. Crude Oil, Brines, and Polymer Solution
A viscous crude oil having a viscosity of around 11 cp at a reservoir temperature of 80 °C was used in the study. The acid number of the oil as measured using colour indicator titration was around 4.3 mg KOH/g of oil. Brine having a salinity of 180k ppm was used as formation water (FW). High salinity brine (HSW) of 13k ppm salinity was prepared using South Caspian Seawater composition and was used for conventional waterflooding. Ion-tuned EW was prepared by diluting HSW 10 times and increasing the concentration of SO42- ions by 6 times and that of Ca2+ ions by 3 times. This composition of EW was selected as it provided promising results in terms of wettability alteration in a previous study [17]. The EW was used after conventional water flooding and to prepare polymer solutions. The ionic composition of the three brines is given in Table 1.
Table 1. Ionic compositions of FW, HSW, and EW.
Ions |
FW (ppm) |
HSW (ppm) |
EW (ppm) |
Na+ + K+ |
81600 |
3240 |
325 |
Ca2+ |
9540 |
350 |
105 |
Mg2+ |
1470 |
740 |
74 |
Cl- |
90370 |
5440 |
544 |
SO42- |
- |
3010 |
1806 |
HCO3- |
- |
220 |
22 |
Total |
182980 |
13000 |
2876 |
A hydrolysed polyacrylamide (HPAM) polymer named Flopaam 5115 was used in the study at an optimum concentration of 1500 ppm. This polymer was selected because of its higher thermal and salinity tolerance and its better performance compared to some other polymers [18]. The 1500 ppm concentration was chosen as it resulted in the desired polymer viscosity of 14 cp.
2. 2. Rock Samples
For contact angle measurements, nine 1.5-inch diameter pellets cut in semi-circles were obtained from the same limestone cores used in coreflood experiments. Three core samples having a 1.5-inch diameter and 3-inch length were cut from Indiana limestone and were used for coreflooding at aging times of 0 days, 2 weeks and 1 month, respectively. The porosities and absolute permeabilities for the three cores were in the range of 14.6-15.0% and 103-134 md, respectively.
2. 3. Contact Angle Measurements
To confirm that the designed EW is capable to recover additional oil by changing the carbonate rock surface towards more water-wet, contact angle (CA) measurements were performed. The main purpose of CA measurements was to evaluate the effect of initial rock wettability on the EW wettability alteration mechanism. Another objective of CA measurement was to confirm that the selected polymer does not affect rock wettability or EW performance. OCA 15EC contact angle apparatus by DataPhysics Instruments GmbH was used and CA was measured by the captive bubble method. In this method, the pellet is placed into the brine and/or polymer solution and an oil drop is injected by a syringe from the bottom of the pellet. The oil drop rises due to the density difference between oil and water and attaches to the rock surface. The oil-water CA is then computed once the oil drop is stabilized. The CA apparatus is schematically presented in Figure 1.
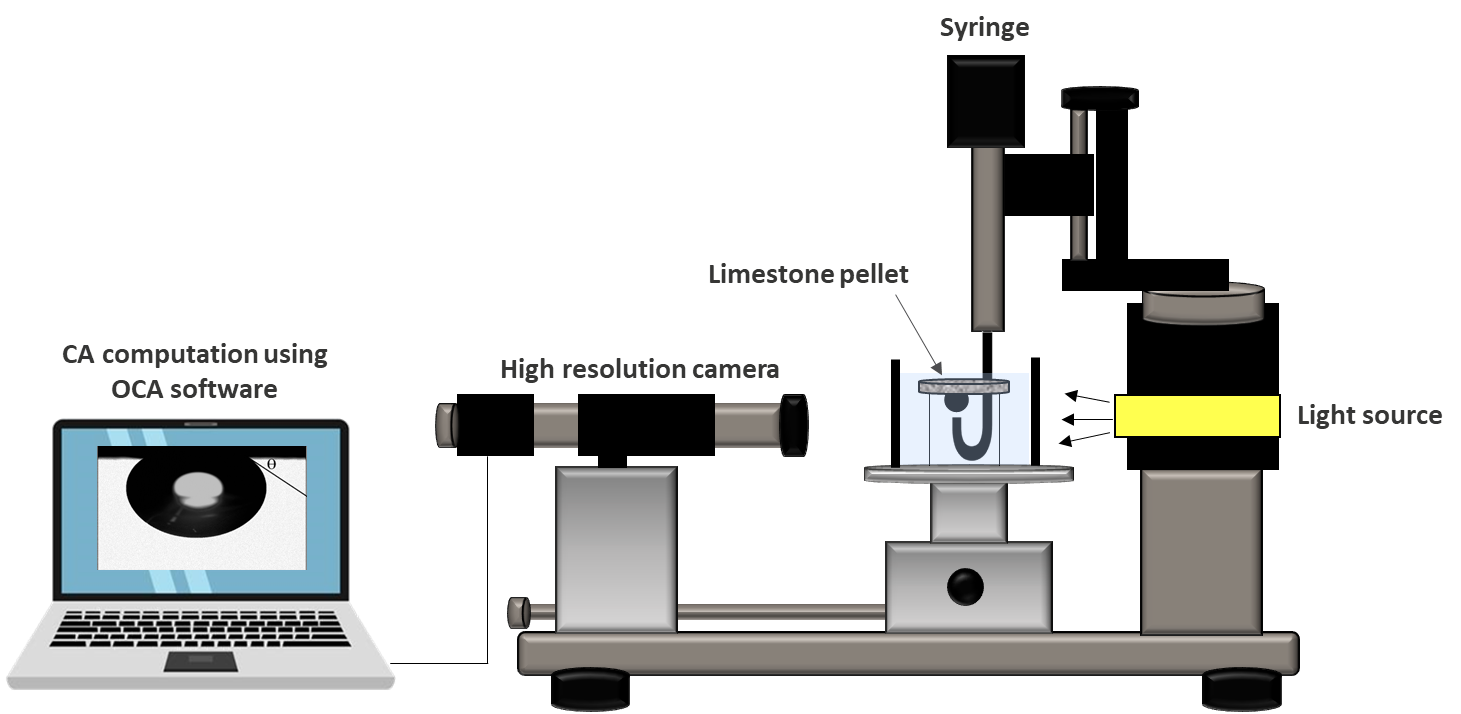
9 pellets were used for CA analysis. All the pellets were first saturated with FW using Vinci manual saturator and the pre-aging CA of the carbonate rock was measured. The pellets were then kept in the crude oil in a special aging cell and placed in the oven at 80 C to restore initial reservoir wettability where the aging time was set as 1 week, 2 weeks, and 1 month for different pellets. After the specified aging time, a set of three pellets was taken out of the oven, and their post-aging CAs were measured. The three pellets were then immersed in HSW, EW at room temperature, and EW at 80 C, respectively, for one week. Here the objective was to compare EW and HSW and to assess the effect of temperature on EW performance to change the rock wettability. One of the 9 pellets with an aging time of one month was placed in the engineered water/polymer (EWP) solution for one-week post-EW-exposure and the CA was again measured to check the effect of polymer on wettability. For better accuracy of results, the CA measurement for each case was repeated at least three times and the average value was used for further analysis and reporting. CA was measured for both top and bottom surfaces of a pellet to check the consistency of the results.
2. 4. Coreflooding
For aging and subsequent coreflooding, each core sample was first saturated with FW using Vinci manual saturator and then flooded with FE using Vinci aging cell apparatus. Oil was injected into the core until the effluent water cut was less than 1% and initial water saturation (Swi) was calculated using water production data. The core was then placed in the oven for the specified time for raging. Afterward, oil was again injected to ensure the core was at Swi. HSW was then injected to obtain oil recovery by conventional waterflooding. EW injection was then started, and the effluent oil production was used to calculate oil recovery and Sor at the end of EWF. The core was then flooded with EWP solution at different rates until there was no more oil production. An EW-Postflush was performed to recover the mechanically trapped polymer. The results were used to obtain recovery factors and Sor after different injection stages and the effect of wettability was studied.
3. Results and Discussion
This section presents the results of the study. Contact angles were measured to quantitatively assess the effect of aging time on EW and hybrid EWPF performance. The pre-aging CA for each pellet was measured and was found to be around 10°-20°, indicating a water-wet medium. Post-aging CAs were then measured after 1 week, 2 weeks, and 1 month of aging time to analyse the effect of aging time.
3. 1. Effect of Aging Time on Initial Wettability
On average, the contact angles measured after 1 week, 2 weeks, and 1 month aging time were 134°, 158°, and 160°, respectively. The measurements were repeated three times and the average values were used for further analysis. As the aging time in crude oil increased, the rock surface changed towards strongly oil-wet after 1 month. This is because the carbonate surface, being positively charged, has an affinity for negatively charged acidic components in the crude oil. As more time was given for these pellets to contact the oil, almost the entire surface strongly became oil wet. Another reason was the high acid number of the crude oil used in this study, leading to more acidic components available for adsorption on the carbonate surface. Overall, these results strengthen the point that carbonate reservoirs tend to be oil-wet because the contact angle changed considerably and quickly shifted from a more water-wet to a more oil-wet state within one week of aging with crude oil.
3. 2. Effect of Initial Wettability on EW Performance
After measuring the post-aging CAs for each set of pellets, they were kept in respective fluids (HSW, EW) for one week and the final CA was measured to see the change. The contact angles were measured using the captive bubble method and the profiles obtained for HSW and EW were compared with the initial profiles. Figure 2 shows the comparison of contact angles at different aging times, and it can be observed that EW resulted in higher contact angle shift compared to HSW.
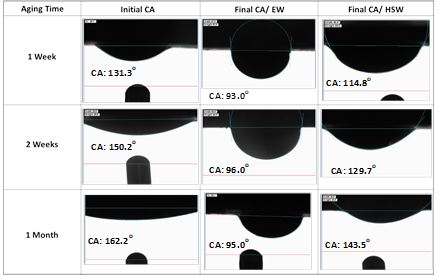
The HSW could only slightly change CA after one month of aging time. Figure 3 shows the relationship between aging time and change in CA by EW and HSW. As the aging time became more, the change in CA caused by EW also increased because of higher adsorption of crude oil acidic components on calcite surface after one-month aging. The change in contact angle by EW was more in the case of 2 weeks and 1-month aged pellets. The oil-wet conditions seem to be more favourable for EW to perform its action of wettability alteration as it is easier to detach the larger number of oil components adhered to the surface by disturbing the initial equilibrium state of the surface. HSW was not much efficient in altering the rock wettability in comparison to EW. It can be related to a high concentration of monovalent and divalent ions present in HSW hindering the oil desorption from the calcite surface. On average, the EW reduced the contact angle by 40-55 degrees, making the rock less oil wet. The efficiency of engineered water in altering rock wettability was around 70% higher than high salinity water, making it a profitable choice for oil-wet carbonate rocks. The positive and negative error bars in Figure 3 indicate the highest and lowest CA change for three repeated measurements on the same pellet.
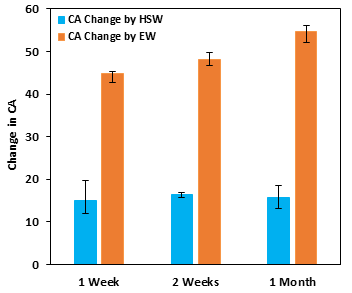
3. 3. Effect of Temperature on Wettability Alteration by EW
At this stage, the effect of temperature on EW extent of wettability modification was also evaluated by keeping one pellet from each set at 80 C. The effect of temperature on EW performance is graphically elaborated in Figure 4.
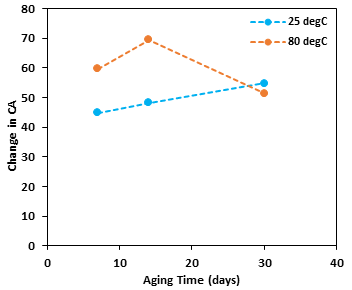
It shows that high-temperature conditions were more effective in changing the CA towards water-wet conditions compared to ambient conditions. After one week of aging, the EW at 80 °C reduced the CA by 60° while EW at room temperature was able to drop the CA by 44°, almost 27% less efficient compared to high-temperature conditions. This trend can be credited to increased activity of PDIs, particularly SO42- in EW at elevated temperature, as it becomes easier for these ions to substitute carboxylic oil components from the rock surface because Mg2+ ions have a higher affinity for SO42- and can displace Ca2+ ions under these conditions [19]. Another contributor to this higher wettability change is the higher calcite dissolution because the rate of mineral dissolution reactions also increases with temperature [20, 21]. However, after one month of aging in crude oil, the change in wettability at high temperature was not as effective as at room temperature. This could be due to a stronger adhesion force between crude oil and carbonate surface after being exposed to oil for one month at 80 °C. In this case, it was difficult for EW to dislodge the oil components and hence the wettability shift was relatively smaller.
3. 4. Effect of Polymer on EW Performance
To check if polymer has any effect on EW capability to alter rock wettability, one pellet was placed in EWP solution while another pellet was placed in EW only and the CAs were measured and compared after one week. The results, as presented Figure 5, show that F5115 had no negative impact on EW performance, because the change in CA by EW and EWP was almost same for both the pellets. EW reduced the CA by 30° while EWP reduced it by 28°. It can be said that this CA change was caused by EW, not by the polymer. Al Sofi et al. [8] also measured CAs for LSW and LSP and concluded that polymer had no adverse influence on CA
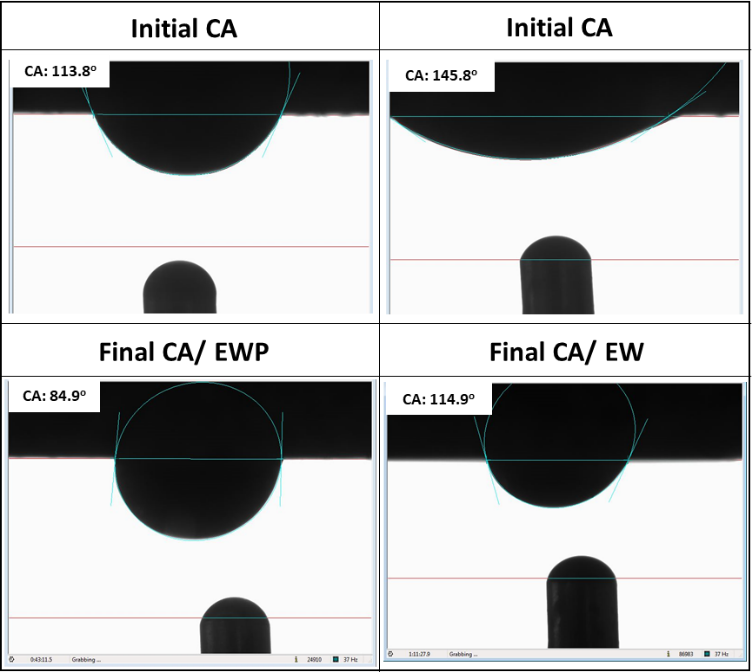
3. 5. Results of Coreflood Experiments
The analysis of the three coreflood tests shows that for water-wet systems, the HSW combined with PF can result in comparable oil recovery as with EWPF, thus hybrid EWPF may not be an optimum choice for such systems. However, in medium to strong oil-wet reservoirs, the Sor after HSW flooding is quite high and EWF and EWPF provide very good incremental recoveries under these conditions. The incremental recovery factors for the three EWPF experiments having different initial rock wettability are given in Table 2. The results indicate that engineered water can recover the residual oil only in intermediate to strong oil-wet reservoirs. The synergetic behaviour of EW and PF is also pronounced under these conditions, particularly in the intermediate oil-wet case. This can be explained by the ability of EW to change the contact angle between oil and carbonate surface. In the case of very weak oil-wet rock, maximum moveable oil is already produced by HSWF, and EWF cannot recover residual oil by wettability change as the rock already has an affinity for water.
Table 2. Summary of oil recoveries by hybrid EWPF under different initial wettability conditions.
Process |
HSW |
EW |
EWPF |
Total |
|
EWPF (Weak Oil-Wet Case) |
RF (%OOIP) |
75 |
76 |
88 |
- |
Inc. RF |
- |
1 |
12 |
13 |
|
RF (%ROIC) |
75 |
4 |
49 |
- |
|
EWPF (Intermediate Oil-Wet Case) |
RF (%OOIP) |
50 |
56 |
83 |
|
Inc. RF |
- |
6 |
27 |
33 |
|
RF (%ROIC) |
50 |
12 |
61 |
||
EWPF (Strong Oil-Wet Case) |
RF (%OOIP) |
51 |
59 |
80 |
|
Inc. RF |
- |
8 |
20 |
28 |
|
RF (%ROIC) |
51 |
16 |
50 |
Thus, all the incremental oil produced by EWPF is due to the improved fractional flow and increased viscous forces by the polymer. When EW encounters an intermediate oil-wet surface, it becomes easier for EW to detach the carboxylic oil material as the affinity of oil towards the rock surface is not too strong. As a result, a higher residual oil saturation is present that can be easily displaced by subsequent EWP front. For this reason, a higher incremental recovery is obtained by EWPF in the intermediate oil-wet scenario. The comparison of incremental oil recoveries as a function of the extent of the oil-wetting nature of the rock is presented in Figure 6.
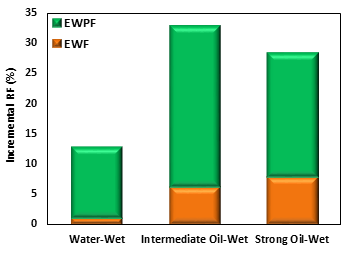
It can be said that EW performed best in strong oil-wet conditions while the hybrid EWPF scenario showed best results in the intermediate oil-wet state. Hence, the optimum wettability state for hybrid EOR methods utilizing EW is intermediate to strong oil-wet conditions. In the third experiment, when EW encountered a strong oil-wet surface, it could not desorb as much oil as it did when the surface was moderately oil-wet. This is because the oil material was strongly clung to the rock surface and a higher change in the surface forces was required to release the oil. Consequently, a relatively lower residual oil saturation was available for EWPF to move out of the core compared to intermediate oil-wet conditions. Hence, EWF is not an optimum EOR choice for water-wet reservoirs and the initial wettability of the system must be taken into consideration during the screening of a reservoir for EWF. The mechanism of EW in different wetting mediums is schematically represented in Figure 7. As aging time increases, the contacted surface area between crude oil and rock also increases, making it difficult to detach the oil droplet from the surface. In Figure 7, L4 > L3 > L2 > L1, indicating that best condition for hybrid EWPF methods is the intermediate oil-wet condition. However, for EWF, both intermediate and strongly oil-wet conditions are favourable.
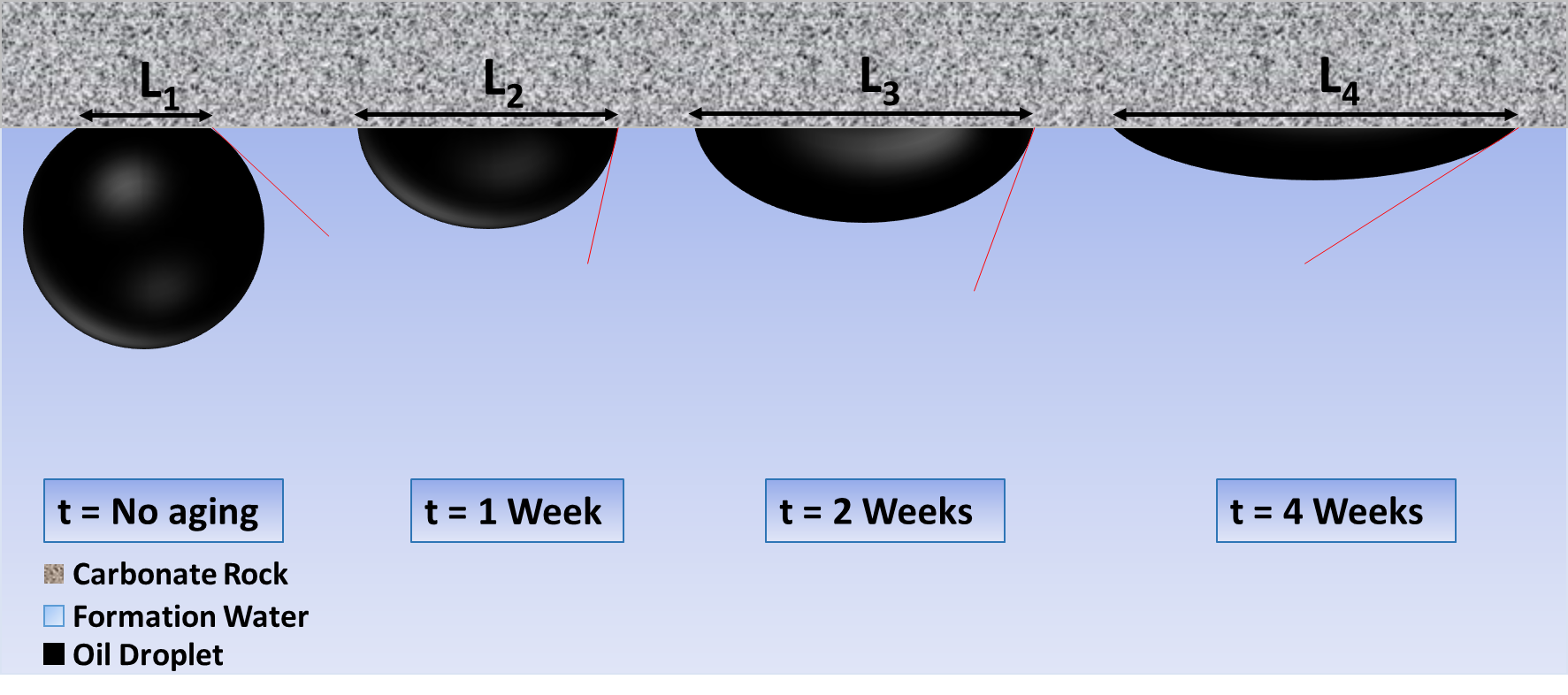
Our work shows that the initial wettability of the system is a critical screening parameter for EW-based enhanced oil recovery techniques. The oil recovery by HSW is 25% less under oil-wet conditions compared to the water-wet case. The recovery by EW is considerably higher in the strongly oil-wet carbonate core (8% of OOIC) as compared to the weak oil-wet core (1% of OOIC). Similarly, the performance of hybrid EWPF is greatly improved under intermediate-wet and strong oil-wet conditions. Capillary numbers are calculated using coreflood data and compared with standalone waterflooding and polymer flooding desaturation curves. Although capillary numbers for three cases are in the same range of 3x10-6 to 4x10-5, the reduction in Sor by EWPF is 16% higher in the strongly oil-wet case compared to the weak oil-wet case.
4. Conclusion
The different factors influencing the recovery of the hybrid EWPF method have been analysed in this research. One of the critical parameters in this regard is initial wettability. The initial wettability of the system is an important factor to be considered for EW-based oil recovery techniques. The contact angle measurements and oil displacement tests carried out in this research study indicate that intermediate to strong oil-wet reservoirs are the best candidate for EWF and hybrid EWPF because of the significant wettability shift caused by EW under these conditions. EWF does not provide any incremental recovery in water-wet or very weak oil-wet reservoirs as the recovery by waterflooding is already quite satisfactory from these reservoirs.
Reservoir temperature is another important parameter to be considered during the screening stage. CA results of this study prove that high-temperature carbonate formations are the best candidates for EW-based EOR techniques. Although EW has caused a wettability shift at ambient conditions as well, however, the CA change is higher at elevated temperatures. This is because EW provides better results at high temperatures due to increased reactivity between PDIs and calcite surface.
Another important factor is the impact of polymer on the EW wettability alteration mechanism. It is confirmed by CA measurements that Flopaam 5115 does not affect EW performance and has no negative role in wettability alteration. Hence, all the factors studied herein confirm the successful synergy between EW and PF by providing higher oil recoveries and increasing the stability of the polymer. The screening criteria for the application of hybrid EW-based methods must, therefore, include the critical parameters discussed herein, including initial wettability, reservoir temperature, and compatibility between the designed EW and the polymer selected for EOR application.
5. Accuracy of Results
The possible sources of error in this study include human error in contact angle measurements. To minimize this error and to increase the accuracy of the results, contact angles are measured at three different locations and measurement at each location is repeated three times. The average of all contact angle values is used in final comparison while highlighting the error bars. Furthermore, the results of contact angle analysis are also verified by coreflood tests as indicated by a low oil recovery by HSW in intermediate oil-wet and weak oil-wet cases. Thus, the results of the study are within the acceptable limits of error and accuracy.
Acknowledgments
The authors would like to thank Nazarbayev University for supporting this research through the NU Faculty Development Competitive Research Grants program (Application of Nanofluids to Control Formation Damage and Improved Oil Recovery Process, Code: 240919FD3928).
References
[1] E. C. Vermolen, M. Pingo-Almada, B. M. Wassing, D. J. Ligthelm, and S. K. Masalmeh, "Low-salinity polymer flooding: improving polymer flooding technical feasibility and economics by using low-salinity make-up brine," in IPTC 2014: International Petroleum Technology Conference, 2014, pp. 1-15. View Article
[2] H. Mohammadi and G. Jerauld, "Mechanistic modeling of the benefit of combining polymer with low salinity water for enhanced oil recovery," in SPE Improved Oil Recovery Symposium, 2012. View Article
[3] H. Alzayer and M. Sohrabi, "Numerical simulation of improved heavy oil recovery by low-salinity water injection and polymer flooding," in SPE Saudi Arabia section technical symposium and exhibition, 2013. View Article
[4] S. Borazjani, P. Bedrikovetsky, and R. Farajzadeh, "Analytical solutions of oil displacement by a polymer slug with varying salinity," Journal of Petroleum Science and Engineering, vol. 140, pp. 28-40, 2015. View Article
[5] A. Santo and A. Muggeridge, "An Investigation into the Benefits of Combined Polymer-Low Salinity Waterflooding," in SPE Asia Pacific Oil and Gas Conference and Exhibition, 2018. View Article
[6] U. Alfazazi, W. AlAmeri, and M. R. Hashmet, "Experimental investigation of polymer flooding with low-salinity preconditioning of high temperature–high-salinity carbonate reservoir," Journal of Petroleum Exploration and Production Technology, vol. 9, pp. 1517-1530, 2019. View Article
[7] M. T. Al-Murayri, D. S. Kamal, H. M. Al-Sabah, T. AbdulSalam, A. Al-Shamali, R. Quttainah, D. Glushko, C. Britton, M. Delshad, J. Liyanage, and R. M. Dean, "Low-Salinity Polymer Flooding in a High-Temperature Low-Permeability Carbonate Reservoir in West Kuwait," in SPE Kuwait Oil & Gas Show and Conference, 2019. View Article
[8] A. M. AlSofi, J. Wang, A. M. AlBoqmi, M. B. AlOtaibi, S. C. Ayirala, and A. A. AlYousef, "Smartwater synergy with chemical enhanced oil recovery: Polymer effects on smartwater," SPE Reservoir Evaluation & Engineering, vol. 22, pp. 61-77, 2019. View Article
[9] Y. Lee, W. Lee, Y. Jang, and W. Sung, "Oil recovery by low-salinity polymer flooding in carbonate oil reservoirs," Journal of Petroleum Science and Engineering, vol. 181, p. 106211, 2019. View Article
[10] R. Khaledialidusti and J. Kleppe, "Significance of geochemistry in single-well chemical-tracer tests by coupling a multiphase-flow simulator to the geochemical package," SPE Journal, vol. 23, pp. 1,126-1,144, 2018. View Article
[11] H. Mahani, A. L. Keya, S. Berg, and R. Nasralla, "Electrokinetics of carbonate/brine interface in low-salinity waterflooding: Effect of brine salinity, composition, rock type, and pH on ζ-potential and a surface-complexation model," Spe Journal, vol. 22, pp. 53-68, 2017. View Article
[12] R. Khaledialidusti and J. Kleppe, "Surface-charge alteration at the carbonate/brine interface during single-well chemical-tracer tests: Surface-complexation model," SPE Journal, vol. 23, pp. 2,302-2,315, 2018. View Article
[13] H. L. Chang, "Polymer flooding technology yesterday, today, and tomorrow," Journal of Petroleum Technology, vol. 30, pp. 1,113-1,128, 1978. View Article
[14] T. Austad, S. Strand, E. Høgnesen, and P. Zhang, "Seawater as IOR fluid in fractured chalk," in SPE international symposium on oilfield chemistry, 2005. View Article
[15] K. R. Gomari, A. Hamouda, and R. Denoyel, "Influence of sulfate ions on the interaction between fatty acids and calcite surface," Colloids and Surfaces A: Physicochemical and Engineering Aspects, vol. 287, pp. 29-35, 2006. View Article
[16] K. Al-Nofli, P. Pourafshary, N. Mosavat, and A. Shafiei, "Effect of initial wettability on performance of smart water flooding in carbonate reservoirs—an experimental investigation with ior implications," Energies, vol. 11, p. 1394, 2018. View Article
[17] A. Sekerbayeva, P. Pourafshary, and M. R. Hashmet, "Application of anionic Surfactant\Engineered water hybrid EOR in carbonate formations: An experimental analysis," Petroleum, 2020/10/20/ 2020. View Article
[18] D. Karimov, M. R. Hashmet, and P. Pourafshary, "A Laboratory Study to Optimize Ion Composition for the Hybrid Low Salinity Water/Polymer Flooding," in Offshore Technology Conference Asia, 2020. View Article
[19] P. Zhang and T. Austad, "Wettability and oil recovery from carbonates: Effects of temperature and potential determining ions," Colloids and Surfaces A: Physicochemical and Engineering Aspects, vol. 279, pp. 179-187, 2006. View Article
[20] G.-Q. Tang and A. Kovscek, "An experimental investigation of the effect of temperature on recovery of heavy oil from diatomite," SPE Journal, vol. 9, pp. 163-179, 2004. View Article
[21] W. Fu, "Modeling of temperature effect on low salinity waterflooding," 2015.