Volume 9 - Year 2022 - Pages 31-37
DOI: 10.11159/jffhmt.2022.004
Theoretical and Experimental Investigation of Mass and Heat Transfer in the Drum in Household Heat Pump Laundry Dryers
Gökhan Sır1, Prof. Şevket Özgür Atayılmaz2
1Arçelik A.Ş. Central R&D
34950 Tuzla, Istanbul, Turkey
gokhan.sir@arcelik.com; atayil@yildiz.edu.tr
2Yıldız Technical University, Department of Mechanical Engineering
YTÜ Makine Fak. Makine Müh. Böl. Merkez Yerleşim E1 Blok No:25 Istanbul, Turkey
Abstract - In addition to the increasing environmental awareness in Europe, Eco-design requirements and related legal regulations increase the competition in the durable consumer goods market. From the point of view of domestic dryers, the increase in energy efficiency has accelerated with the integration of the heat pump system into domestic tumble dryers. The price competitive design of these systems makes it important to model these systems and bring them to the most appropriate performance level. In this study, the effects of air flow, drum speed, drying capacity and compressor speed on heat and mass transfer in the drum were investigated in domestic heat pump dryers. Tests were carried out at 3 different load types, drum speed, air flow and compressor speed. As a result of the tests, the air and mass transfer coefficients in the drum were calculated. It has been observed that the parameter that most affects the heat and mass transfer coefficient in the drum is the load capacity. Load capacity is followed by compressor speed, air flow rate and drum speed, respectively.
Keywords: heat pump, laundry dryers, heat transfer coefficient, mass transfer coefficient
© Copyright 2022 Authors - This is an Open Access article published under the Creative Commons Attribution License terms Creative Commons Attribution License terms. Unrestricted use, distribution, and reproduction in any medium are permitted, provided the original work is properly cited.
Date Received: 2022-05-10
Date Accepted: 2022-05-20
Date Published: 2022-06-01
1. Introduction
Energy consumption is of critical importance in the developing world economy. With the application of heat pump systems in drying machines, an average of two times less energy consumption is achieved compared to electric drying systems. However, studies are needed to reduce energy consumption in heat pump drying systems. The most complex process in drying machines is the examination of the movement of textiles in the drum.
Yuhui Wei et al. investigated the effect of different textile type, rotational drum speed, and amount of textile on drying performance. Energy consumption, drying time, textile wrinkle value and textile abrasion values were investigated as drying performance [1]. Xinchen Yu et al. investigated the transverse motion of fabrics in domestic tumble dryers under different drying conditions. They used a high-speed detection system to track of fabric movement in drum. Their experimental results showed that fabric motion is complicated and can be affected by the size of fabrics as well as by drying load mass [2]. A mathematical modeling study that simulates the heat and mass transfer from the core to the porous fabric was carried out in ventilation dryers by Yuhui Wei et al [3]. Lovrenck Novak et al. are investigated to how the inlet air temperature, relative humidity, and flow rate influence the textile drying process in an open cycle tumble dryer [4]. Chris Jones et al. examined the dynamics of motion within the dryer across a range of movements experienced by items during tumbling. They used positron emission particle tracking system to analyses the motion of radioactively labeled tracer particles attached to fabrics and solid fabric enhancer delivery articles in tumble dryers. The most significant changes in this behavior were observed with changes in fabric moisture content and volumetric fill ratio, with moist fabric and smaller load sizes both exhibiting faster fall velocities and spending more time in the fabric bed [5]. In the study carried out by Pero Gataric et al., the energy consumption and drying time of the heat pump dryer were modeled. As a result of the study, it has been seen that the interaction of textiles with drying air in a rotating drum is of great importance for increasing energy efficiency. Increasing the mass load at a fixed drum volume increases the contact area between the textiles and the drying air, which increases energy efficiency. This can be explained by the relationship between the contact area and the rate of heat and mass transfer. A large contact area increases the heat transfer from the drying air to the textile surface and provides greater moisture transfer from the textile surface to the drying air. If the mass load is too small, it is possible that the air is unsaturated when leaving the drum due to poor contact with the textiles [6]. Philip Boudreaux et al. are investigated on where air leaks occur in the system in dryers. In the studies, two vented and one closed system textile dryer were used. As a result of the experimental studies, it has been observed that the system has a 60% of the leaks at the fan outlet, and it has been observed that the effect of these air leaks on the system performance is negligible [7]. Kyle Gluesenkamp et al. are studied on heat and mass transfer efficiency in vented type dryers were carried out. The correlations found in this study were produced with four measurable values. These values were determined as drying air flow rate, load amount, air mass in the drum and the drop time of the full load in the drum. These parameters are dimensioned using the Buckingham Pi theorem [8].
In this study, the effects of compressor speed, drum speed, load capacity, air flow rate on mass and heat transfer in the drum were investigated in heat pump drying systems with variable speed compressor.
2. Experimental Setup
It is aimed to examine the heat and mass transfer change in the drum by measuring the moisture amount and temperatures removed from the textile in the cycle. In order to understand the fan, motor and variable speed compressor speeds and the dehumidification process, a test setup was created to collect temperature, humidity and flow rate data from the necessary points.
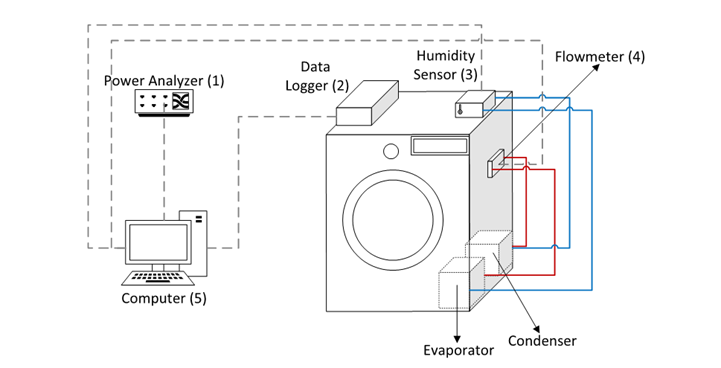
In contrast to the standard dryers, two motors were used to control the drum speed and air flow, which were determined as parameters in the experimental setup. In standard dryers, both the drum and the fan are driven by a motor. For this reason, the fan and the drum rotate at the same motor speed.
Table 1. Test parameter
Load Capacity [kg] |
Drum Speed [rpm] |
Air Flow Rate [lt/sn] |
Compressor Speed [rpm] |
2 |
42 |
48 |
2100 |
5 |
52 |
58 |
2400 |
8 |
62 |
68 |
3000 |
The test parameters are indicated in Table 1. Tests were carried out at 3 different load types, drum speed, air flow and compressor speed. As a result of the tests, the air and mass transfer coefficients in the drum were calculated. The textiles used in the experiments were prepared in accordance with the European dryer standard EN 61121 [9].
2. 1. Measurement System
A data logger system was used to collect temperature data. Temperature data were taken from both the evaporator and the condenser as well as the air passage points. T type thermocouples and KEYSIGHT 34970A data logger were used to measure temperature and collect data. Temperature values were taken from 13 different points on the dryer. 10 of them are placed to measure the refrigerant temperature values at the compressor, evaporator, and condenser, and 4 of them are placed to measure the air temperature values at the drum inlet and outlet, the evaporator inlet and the condenser outlet.
Relative humidity values are needed to calculate the specific humidity to be used in heat and mass transfer calculations. In this experimental setup, a relative humidity sensor was used to receive data from 6 different points from the condenser outlet and the evaporator inlet. Arduino Nano and Coolterm interface were used to collect data from the sensor. The relative humidity, the pressure taken at 1 atm and the temperature values in these areas and the specific humidity values calculated by using the Coolprop library [10] in the python programming language.
Flow measurements in the dryer were carried out with an Arduino Nano and a flow sensor. The system works by connecting two standard pipes coming out of the condenser outlet and between the evaporator and the condenser to the flow sensor, and the pressure difference values read here are read by the arduino and both pressure difference and flow value are displayed on the Agilent VEE Pro interface.
The Sensirion brand flow sensor used in the hybrid dryer where the experiments will be carried out is shown in Figure 2. The sensor has two outputs. One of these outlets is connected to the condenser outlet and the other is connected between the evaporator and the condenser. The sensor measures the pressure difference between these two points.
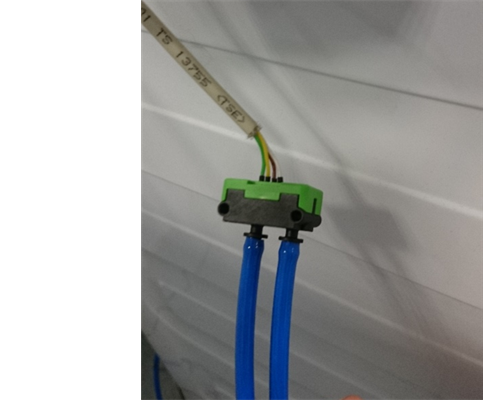
Calibration of the flow sensor is required for accurate flow measurements. For this, calibration was performed by inserting the dryer into the wind tunnel shown in Figure 3.
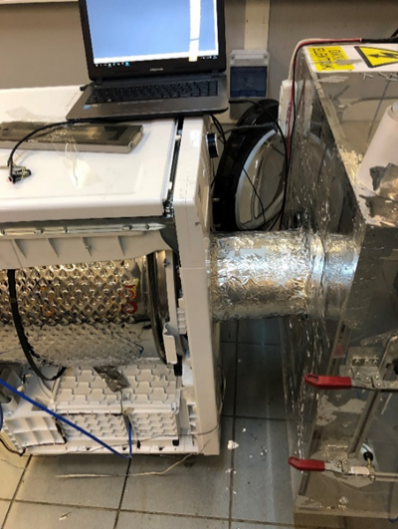
In the tests carried out in the dryer after the wind tunnel calibration, the change in the air flow value was instantly followed.
3. Mathematical Model
In the literature, the relationship between the rate of dehumidification from the laundry in the drum and the mass transfer is shown by Equation 1 [11].

where k is the mass-transfer coefficient, A is total area of the cloth surface, ρv is the water density at the cloth surface, and a is an activity coefficient.
The activation coefficient for cotton type textiles was formulated in the study of Lambert et al. [12]. The activation coefficient equation is shown in Equation 2.
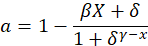
where β,δ and γ are consant. X is specified as the relative humidity value on the textile. As a result of the studies by Lambert et al., the coefficient constants of β, δ and γ were found to be 18, 30 and 2 for cotton type textiles, respectively.
The relationship between the heat and mass coefficient in the drum is shown as Equation 3 in the article published by Yadav et al. in 2008 [13].
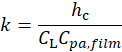
Where hc is the heat transfer coefficient [kW⁄m2 K], Cpa,film is the specific heat value of the air in the textile film [kJ⁄kg K] and CL is the Lewis number. The Lewis number is calculated with the formula shown in Equation 4.
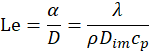
Here, λ is the heat transmission coefficient (kW/mK), ρ is the density (kg/m3), is the diffusion coefficient of the air-water mixture (m2/sec), and is the specific heat of air (kj/kg.K).
The heat and mass transfer coefficients in the drum were calculated by applying these formulas used in the literature.
The following assumptions were made in the modeling studies.
- The thermo-physical properties of the textile material used are the same throughout the volume used.
- The moisture distribution inside the textile material is homogeneous.
- The instantaneous moisture change of the drying fluid is equal.
- The temperature distribution inside the textile material is instantaneously equal.
- The moisture transfer from the drying fluid to the textile material takes place in the specified control volume.
- Determined control volume can be static or mobile, both cases are considered.
- Drying air with low humidity enters at one point and exits at the other.
4. Results
As a result of the tests, the effect of laundry drying capacity, drying air flow rate, drum speed and compressor speed on the heat and mass transfer in the drum was observed.
4. 1. The effect of load capacity on heat and mass transfer coefficient inside the drum
The effect of the tested load capacity on the mass transfer coefficient is shown in Figure 4. In order to see the change in different capacities in the tests performed, the optimum drying cycle time was taken between 20 and 80 minutes.
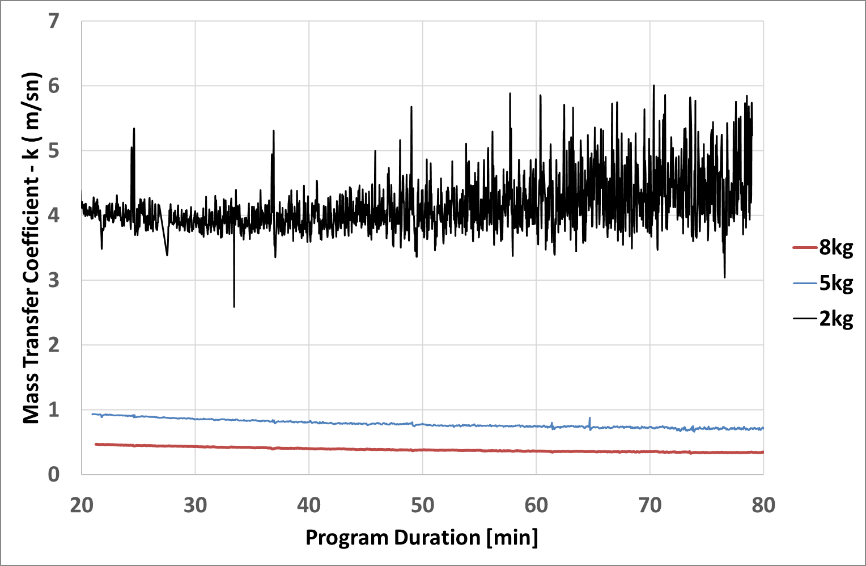
It has been observed that the mass transfer coefficient increases as the load capacity decreases within the fixed drum volume. During the drying process, the mass transfer coefficient within each capacity remains constant.
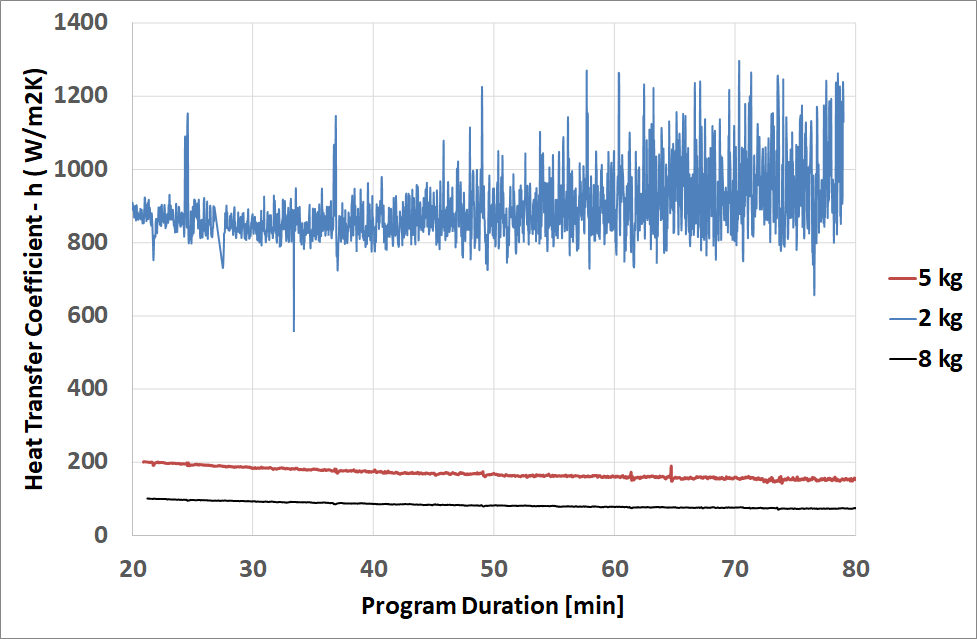
The effect of the tested load capacity on the heat transfer coefficient is shown in Figure 5. Similar to the mass transfer coefficient, the heat transfer coefficient increases with the decrease of the tested load capacity.
4. 2. The effect of air flow rate on heat and mass transfer coefficient inside the drum
By keeping the load capacity, compressor speed and drum speed constant, the effect of air flow change on the mass transfer coefficient inside the drum is shown in Figure 6.
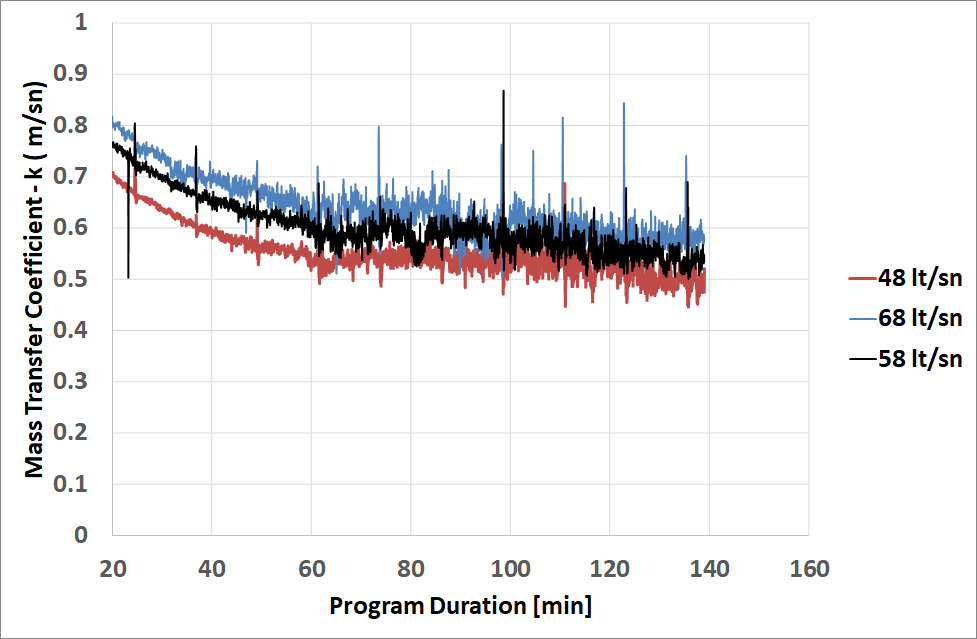
The increase in the flow rate during the drying cycle caused an increase in the mass transfer coefficient. However, after the 60th minute of drying for each flow rate value, it is seen that the mass transfer coefficient remains constant, and the values approach each other. The reason for this is that the evaporation of water on the textile surface in the first phase of drying increases depending on the flow rate. For this reason, it has been observed that the mass transfer coefficient of moisture on the laundry is directly proportional to the flow rate. Towards the last phases of drying, it is necessary to evaporate the water in the inner structure of the textile. In this case, the increase in flow does not show the expected increase in mass transfer.
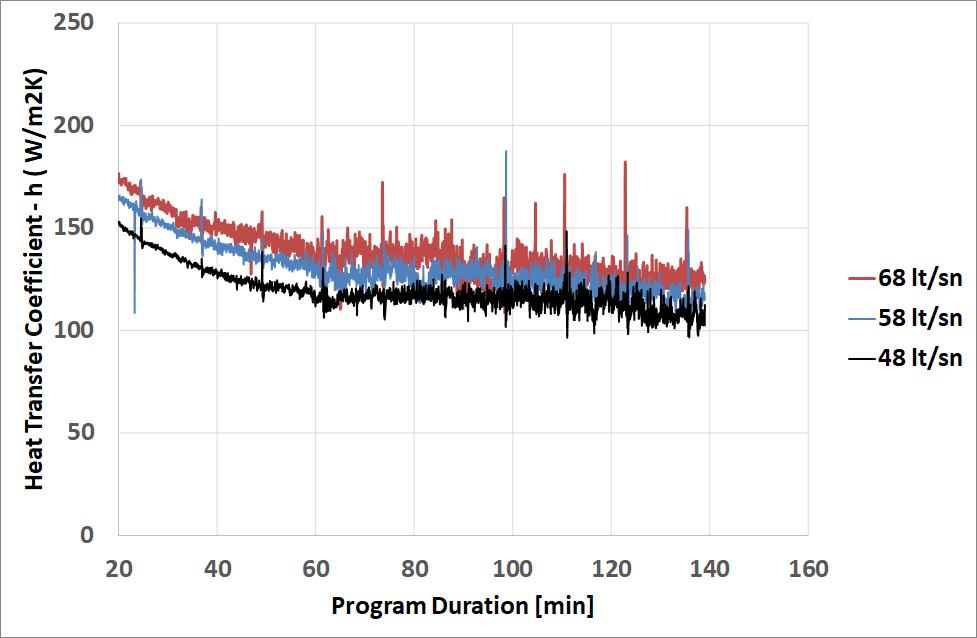
The effect of the air flow rate on the heat transfer coefficient is shown in Figure 7. Similar to the mass transfer coefficient change phenomenon, it is observed that the heat transfer coefficient also changes.
4. 3. The effect of drum speed on heat and mass transfer inside the drum
The effect of the drum speed change on the mass transfer coefficient in constant load capacity, compressor speed and air flow rate are shown in Figure 8.
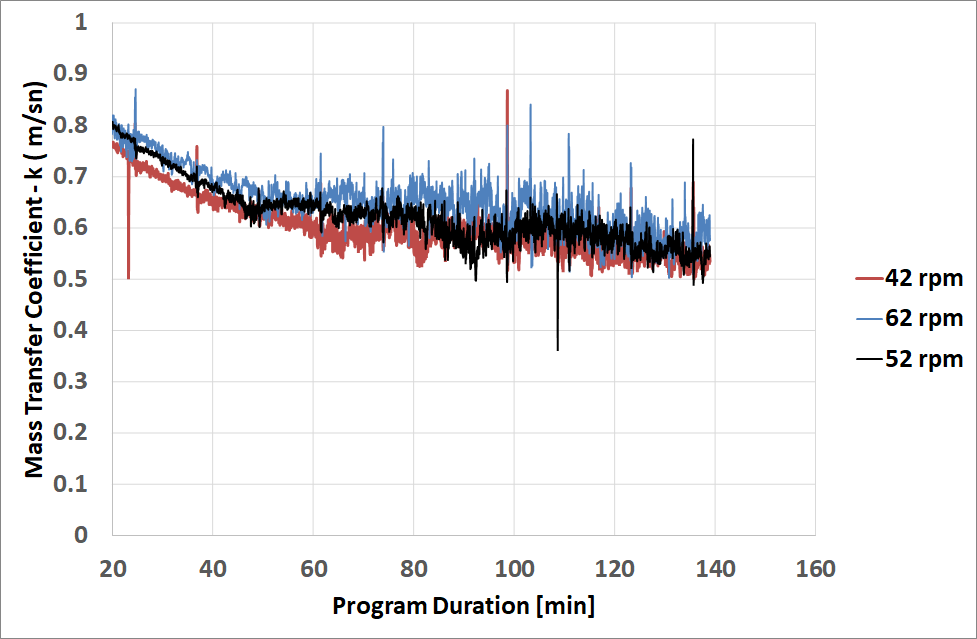
It has been observed that the increase in drum speed increases the mass transfer coefficient, albeit partially. In parallel with the air flow rate change, the effect of the change in the drum speed on the mass transfer coefficient decreases towards the final phases of drying.
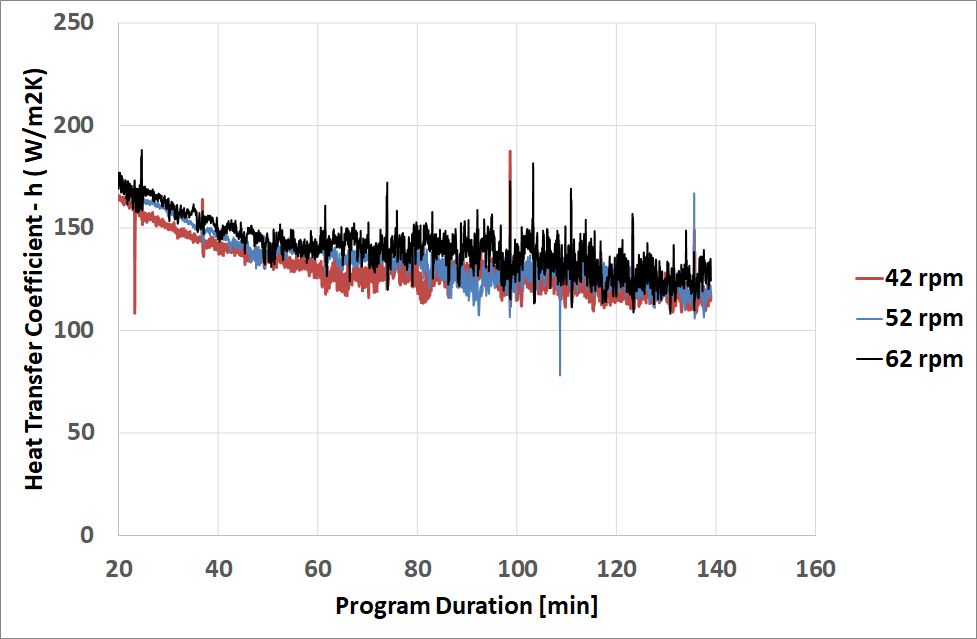
The effect of drum speed on the heat transfer coefficient is shown in Figure 9. The increase in drum speed in parallel with the mass transfer coefficient caused a partial increase in the heat transfer coefficient.
4. 4. The effect of compressor speed on heat and mass transfer inside the drum
The effect of variable speed compressor speed on the mass transfer coefficient is shown in Figure 10.
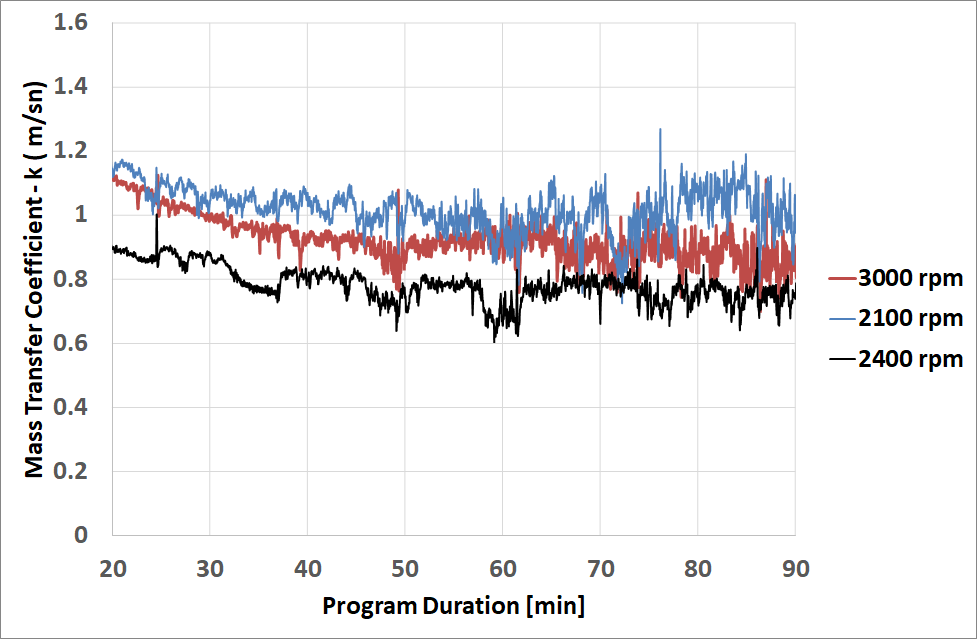
The highest mass transfer coefficient value was reached at 2100 rpm compressor speed. Inverter compressor speed directly affects the heating and cooling capacity in the system. In the heat pump drying system, the heating capacity increases as the compressor speed increases. However, since the flow in the system goes directly to the condenser, the amount of refrigerant in the evaporator, where dehumidification takes place, has decreased, and accordingly, the dehumidification capacity has decreased. For this reason, the minimum mass and heat transfer value was observed at 3000 rpm compressor speed. It was observed that the highest mass transfer coefficient in constant load capacity, drum speed and air flow rate was realized at 2100 rpm compressor speed.
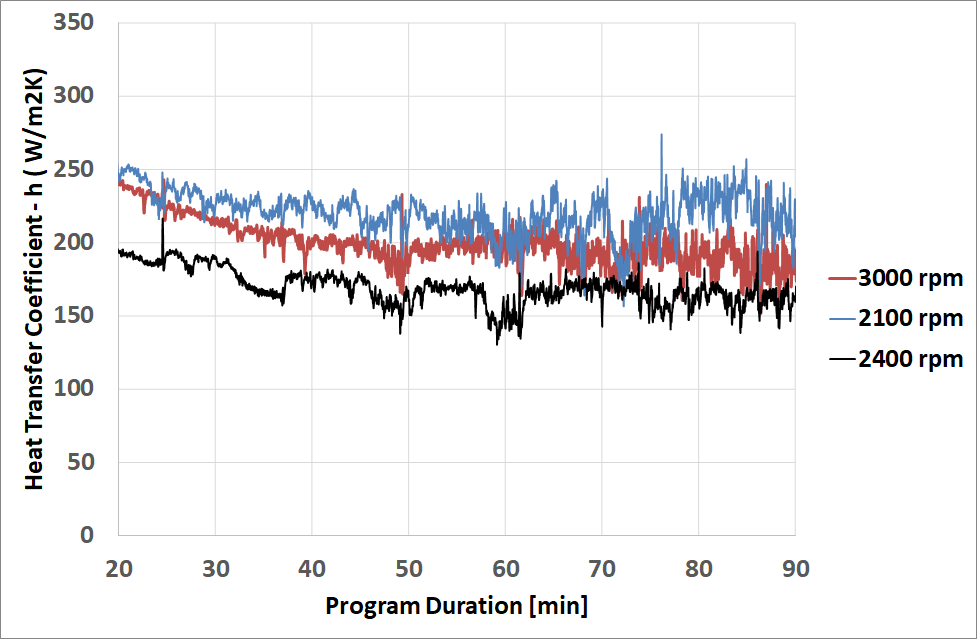
The effect of compressor speed on the heat transfer coefficient is shown in Figure 11. The results obtained were found to be like the mass transfer coefficient.
5. Conclusions
In this study, the effects of compressor speed, drum speed, load capacity, air flow rate on mass and heat transfer in the drum were investigated in heat pump drying systems with variable speed compressor.
- It has been observed that the parameter that most affects the heat and mass transfer coefficient in the drum is the load capacity. Load capacity is followed by compressor speed, air flow rate and drum speed, respectively.
- The highest mass and heat transfer coefficient values were achieved in tests with a capacity of 2 kg.
- The increase in the flow rate during the drying cycle caused an increase in the mass and heat transfer coefficient. However, after the 60th minute of drying for each flow rate value, it is seen that the mass transfer coefficient remains constant, and the values approach each other.
- The increase in the drum speed during the drying cycle caused an increase in the mass and heat transfer coefficient. The changes in mass and heat transfer coefficient were observed in parallel with the volume flow rate.
- Inverter compressor speed directly affects the heating and cooling capacity in the system. The heating capacity increases as the compressor speed increases. However, since the refrigerant flow in the system goes directly to the condenser, the amount of refrigerant in the evaporator, where dehumidification takes place, has decreased, and accordingly, the dehumidification capacity has decreased. In this experimental study, it was observed that the most efficient compressor operating speed was 2100 rpm.
Acknowledgements
This research was supported by Arçelik.
References
[1] Wei, Y., Su, Z., Zhang, Y., Li, P., & Yuan, H. (2019). The effect of fabric movement on drying performance of the domestic drum dryer. The Journal of The Textile Institute, 110(7), 1059-1071. View Article
[2] Yu, X., & Ding, X. (2021). The transverse motion of fabrics in domestic tumble dryers under different drying conditions. Drying Technology, 39(1), 35-51. View Article
[3] Wei, Y., Gong, R. H., Ning, L., & Ding, X. (2018). Enhancing the energy efficiency of domestic dryer by drying process optimization. Drying Technology, 36(7), 790-803. View Article
[4] Novak, L., Gatarić, P., & Širok, B. (2019). Influence of drum inlet air conditions on drying process in a domestic tumble dryer. Drying technology, 37(6), 781-792. View Article
[5] Jones, C. R., Corona, A., Amador, C., & Fryer, P. J. (2021). Dynamics of fabric and dryer sheet motion in domestic clothes dryers. Drying Technology, 1-18. View Article
[6] Gatarić, P., Širok, B., Hočevar, M., & Novak, L. (2019). Modeling of heat pump tumble dryer energy consumption and drying time. Drying technology, 37(11), 1396-1404. View Article
[7] Boudreaux, P., Gluesenkamp, K. R., Patel, V. K., & Shen, B. (2021). Measurement and analysis of clothes dryer air leakage. Drying Technology, 39(14), 2105-2117. View Article
[8] Gluesenkamp, K. R., Boudreaux, P., Patel, V. K., Goodman, D., & Shen, B. (2019). An efficient correlation for heat and mass transfer effectiveness in tumble-type clothes dryer drums. Energy, 172, 1225-1242. View Article
[9] EN 61121, (2013), Tumble dryers for household use - Methods for measuring the performance, EN 61121
[10] Coolprop library, http://www.coolprop.org/citation.html#citation-for-coolprop
[11] Williamson NJ. Energy efficiency of commercial-laundry dryers. Project in mechanical engineering. The University of Auckland; 2002.
[12] Lambert, A. J. D., F. P. M. Spruit, and J. Claus. "Modelling as a tool for evaluating the effects of energy-saving measures. Case study: a tumbler drier." Applied Energy 38.1 (1991): 33-47. View Article
[13] Yadav, V., and C. G. Moon. "Fabric-drying process in domestic dryers." Applied energy 85.2-3 (2008): 143-158. View Article