Volume 8 - Year 2021 - Pages 238-243
DOI: 10.11159/jffhmt.2021.025
Unlocking the Future with Flexible, Wire-Shaped Supercapacitors
Joe Sleppy1, Alisa Silverstein3, Deon Moser3
1Capacitech Energy, Inc.
3259 Progress Drive, Orlando, FL 32826
joe.sleppy@capacitechenergy.com
Abstract - Supercapacitors are critical to the mainstream adoption of a variety of technologies enabling applications such as Cleantech, Wearables, and Internet of Things (IoT). While the value added by supercapacitors is known and well documented, finding the physical space to incorporate supercapacitors in those systems is a challenge to overcome.
Traditional supercapacitors are physically rigid energy storage components that are available in a cylindrical or rectangular shape that are installed on printed circuit boards (PCBs), where space is highly valuable. This traditional form factor limits an engineer’s placement options making it difficult to utilize supercapacitors in certain applications. This physical restriction forces engineers to make difficult design and performance tradeoffs.
Capacitech Energy, Inc. is commercializing research from the University of Central Florida that reimagined the form factor of supercapacitors to be physically flexible and wire-shaped. This innovation leverages its novel form factor to eliminate the need to make painful engineering tradeoffs. Flexible supercapacitors can be routed through areas where space is available on the PCB or alternatively, placed off the PCB and integrated into other parts of the product or system’s infrastructure. This placement advantage and the supercapacitor’s high-power density make this technology well suited to complement modern energy harvesting technologies and batteries used in a variety of applications.
Many opportunities exist to complement power sources with this cable-based supercapacitor’s peak-power capability and to build cable-based backup power systems. This innovation helps designers meet their goals by building discrete and distributed networks of supercapacitors, made possible by this innovative form factor helping engineer build next generation smart city electronics, wearables, solar power systems, electric vehicles and more.
Keywords: Energy storage systems, supercapacitors, internet-of-things, wearables, flexible electronics
© Copyright 2021 Authors - This is an Open Access article published under the Creative Commons Attribution License terms Creative Commons Attribution License terms. Unrestricted use, distribution, and reproduction in any medium are permitted, provided the original work is properly cited.
Date Received: 2021-08-20
Date Accepted: 2021-08-27
Date Published: 2021-09-28
1. Introduction
Traditional supercapacitors play an important role in energy storage systems [2] and are designed to:
- Charge and discharge a lot of energy very quickly due to their low impedance, which is characterized by their high-power density.
- Feature a long cycle life.
- Be safe and less sensitive to current fluctuations within their voltage rating.
However, their adoption is limited as the addition of supercapacitors in many designs come with painful tradeoffs. These tradeoffs are largely due to (1) having an energy density lower than that of a battery, (2) featuring a low voltage rating (2.7V) per unit requiring many units connected in series or parallel to meet application requirements, and (3) their rigid form factor commonly bound to printed circuit boards (PCBs). In summary, a lack of available space in an application limits supercapacitor adoption.
Supercapacitor are constrained by the size, weight, and power constraints (SWAP-c) that restrict energy storage systems. The Cable-Based Capacitor (CBC) innovation is a wire-like, physically flexible supercapacitor. Aside from its coaxial cable and mechanically flexible construction, the CBC functions and operates like a traditional supercapacitor [1].
The energy storage industry has generally focused on material science innovations to improve electrode and electrolyte materials. While this is incredibly important work, the CBC’s form factor innovation addresses the lack-of-space challenge faced by traditional supercapacitors. The CBC is designed to optimize designs, improve performance, and add features by helping engineers overcome tradeoffs typically faced when using various supercapacitors.
2. Form Factor Advantages
The chief advantage of the CBC is its form factor, which enables supercapacitors to be used in new ways and in new places. A flexible supercapacitor can go where supercapacitors are needed but cannot presently be installed.
2.1 Saving Space on Printed Circuit Boards
Traditional supercapacitors are installed on PCBs or are grouped in modules that can be plugged into larger systems. As shown in Figure 1 a supercapacitor is often the largest component on the PCB, consuming valuable surface area on the circuit.
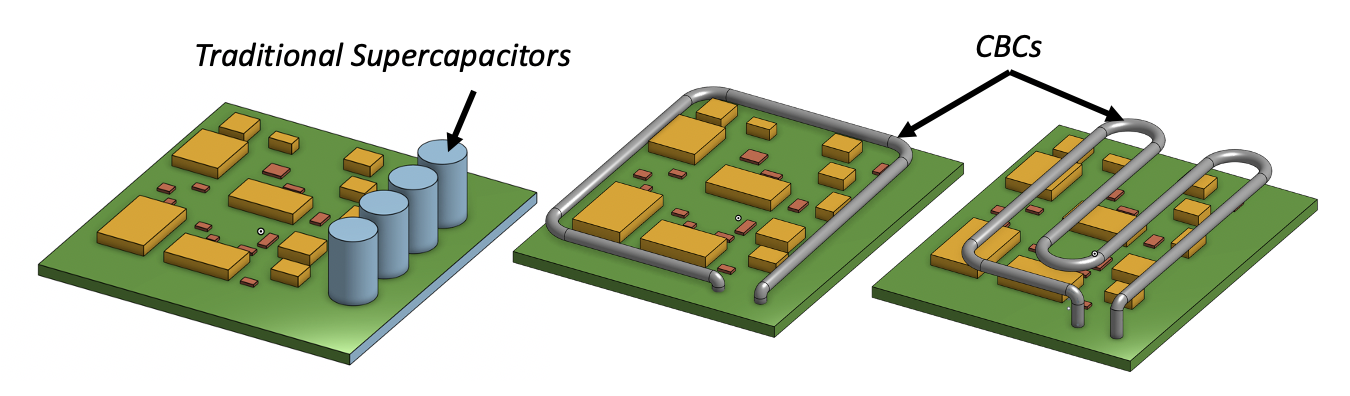
In the example shown in Figure 1, using CBCs in place of traditional supercapacitors made the PCB ~20% smaller by bending CBCs into free space. This enables an engineer to miniaturize the circuit design or add new features in the unused area on the circuit board.
While flexibility lets the CBC be bent into small enclosures and routed through free space on the PCB, it also saves space by the way it connects to the PCB. Traditional supercapacitors have radial bodies that look like a soda can. The cross-sectional area of this radial component is equal to the surface area required on the circuit board. As a result, there is a tradeoff between the height of the supercapacitor and its cross-sectional area. A taller supercapacitor may have a smaller cross-sectional area but will require a larger housing enclosure. A shorter supercapacitor of the same capabilities will be shorter but have a much larger cross-sectional area occupying more space on the PCB.
2.2 Discrete & Distributed Networks of High-Power Energy Storage
Since supercapacitors are designed to be installed on PCBs or connected as modules installed in a system, they are typically found in concentrated groups. Alternatively, the CBC could be integrated into a product or system’s infrastructure to build a discrete and distributed network of power dense energy storage. This placement advantage would address the SWAP-c challenges typically faced by traditional supercapacitors (concentrated on PCBs or inside modules) by distributing them throughout the system.
Supercapacitor applications are generally connected in parallel to input power sources or output loads. Wiring infrastructure is often used to connect those sources and loads together. For this reason, installing the wire-like supercapacitor inside a product or within the system’s wiring infrastructure is a natural design opportunity.
3. Typical Supercapacitor Applications
Supercapacitors are typically used in applications that need additional peak power, load levelling, backup power, or energy storage of intermittent sources.
3.1 Peak Power Function
Power sources are not infinite and loads often need more power than what sources can offer, as shown in Figure 2. This can happen when an Internet of Things (IoT) device turns on their transmitters or an electric motor requires a surge of current when initially turned on.
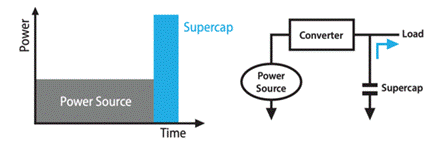
Supercapacitors have high power density due to their low impedance. As such, supercapacitors can be connected in parallel with power sources to inject additional current in short bursts in addition to what the source can provide on its own. This improves performance and reliability of common sources such as batteries and solar cells, providing engineers and designers additional options to consider for their main power supply.
3.2 Unexpected Power Loss.
Power outages can last anywhere between a fraction of a second to hours. Outages that last less than a second are common and can cause a major problem for mission critical equipment (such as telecom and life sustaining equipment), computer memory, leading to long reset times as devices go through protocols to reset power. Figure 3 demonstrates that supercapacitors can respond immediately and provide energy during that glitch, shortage, or outage.
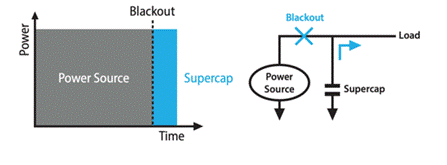
3.3 Storage for Energy Harvesting
Energy harvesting technologies convert one form of energy (light, wind, heat, motion, and even radio frequencies) to an electrical form. These sources may not provide consistent energy as portrayed in Figure 4.
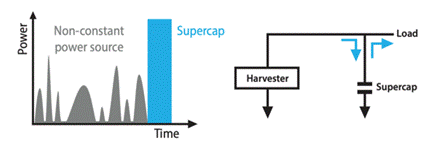
Due to the low impedance that enables their peak power capability and their simple charging mechanism, supercapacitors can easily charge from the intermittent energy from harvesting sources at very low currents.
4. Flexible Supercapacitors as a Complement
As described in the prior sections, supercapacitors have a distinguished set of desirable features and characteristics. Furthermore, flexible supercapacitors address a major hurdle to their adoption. The opportunity is to use the novel form factor of flexible supercapacitors to seamlessly integrate them into systems to unlock the known advantages they offer. Battey-supercapacitor hybrids and energy harvesting-supercapacitor hybrids stand to benefit and can be used to accelerate current technology trends.
4.1 Battery-Supercapacitor Hybrids
Batteries, like supercapacitors, are not all-encompassing perfect solutions. They have many desirable features including a high energy density, near-constant output voltage, and low self-discharge rates. However, batteries are also challenged by a short service life, peak power capacity, temperature sensitivity, and charge/discharge sensitivity. Where the supercapacitor excels, the battery is challenged and vice versa. For this reason, an ideal energy storage system should feature both batteries and supercapacitors.
In a battery-supercapacitor hybrid design, the supercapacitor is positioned to handle fluctuations in load power requirements such that the battery operates under constant charge and discharge conditions. This protects the battery from stresses that can lead to damage and lost performance, which can extend the operating life of the battery.
Traditionally, due to SWAP-c, such a hybrid design would require fewer batteries to be used so that there is space to fit supercapacitors inside the energy storage system. Adding supercapacitors at the expense of batteries will reduce the overall energy storage capacity of the system. This is generally seen as a negative tradeoff. This problem can be addressed with the flexible form factor of the CBC as the supercapacitors are installed in alternative locations, such as the wiring infrastructure, so that supercapacitors can be added without removing batteries from the energy storage system.
When the goal is to maximize operating life and/or charge time it may be acceptable to add supercapacitors and use fewer batteries. This is an opportunity to streamline and miniaturize energy storage system designs. By using fewer batteries and leveraging the CBC’s form factor to install supercapacitors in alternative locations, designers can add new features in the space the previous energy storage system consumed or can make the product or system smaller all together.
4.2 Energy Harvesting-Supercapacitor Hybrids
Solar cells, while not an energy storage source, is growing in popularity as a power source for loads as small as Internet of Things sensors to the power grid. They have a long service life and are well understood by engineering communities in how they operate. Solar cells were traditionally designed for outdoor applications but are increasingly finding their ways indoors to harvest low levels of light. Today, companies like Dracula and PowerFilm offer products operating in dim indoor lighting <200 LUX [3].
Technologically, the solar cell is challenged by the intermittency of light sources it requires to operate and a limited peak performance capacity (based on lighting levels). Pairing solar cells with energy storage technology addresses these challenges. If the light source is covered, the energy storage technology takes over so that the load is not interrupted.
In remote and battery powered IoT applications, the short service life of batteries can be a major problem. As the battery ages, it must be replaced, creating a large burden for the technician managing the IoT system. It is expected that 20% of a technician’s time at facilities with IoT systems will be spent changing batteries [4] making it a high priority to find ways to extend battery life.
Since IoT devices already include batteries in their design, indoor solar cells were used to take over part of the load for the battery when there was sufficient lighting to do so. This successfully extends battery life. Further analysis reveals that remote IoT loads are volatile. When an IoT device transmits data wirelessly, it requires a relatively large current surge to do so. In a solar-battery hybrid design, the solar cell often cannot provide that level of current (power) even under ideal lighting. While a battery may provide the current required, it risks damage doing so.
For these reasons, solar-supercapacitor hybrids are coming into focus. A battery’s operating life does not compare to that of a supercapacitor. Additionally, supercapacitors require very little current to charge, which is perfect for indoor solar cells under a variety of lighting scenarios. In this kind of hybrid design, a solar cell provides the energy to charge the supercapacitor and power the “sleep” state of an IoT device, while the supercapacitor powers the “active” state for wirelessly transmitting data.
This concept is not limited to solar cell technologies but can also be used with other energy harvesting technologies such as radio frequency or vibration energy harvesting [5]. Standing on the shoulders of recent technological progress in harvesting technology, electronics, and supercapacitors, these hybrid designs can replace batteries in certain IoT applications all together, saving technicians from frequent and costly replacements.
5. Battery-Free IoT Fall Alert Use Case
The CBC is particularly useful in applications where comfort, the utilization of space, and power is paramount. The CBC was used in a prototype wearable electronics application that leveraged indoor solar cells (energy harvesting technology).
Wearable electronics are designed to be discrete and integrated with their user, which has driven significant efforts into the miniaturization of electronic devices. The miniaturization of electronic components, such as semiconductors, has significantly outpaced the miniaturization of energy storage components. The utility and capability of a wearable device is largely limited by its energy storage capacity.
5.1 Application Overview
A popular IoT device is used to help the elderly plea for help in case they have fallen. This has led to a demand for fall detectors and SOS pendants that can always be within reach, such as around the neck or wrist. An accelerometer can detect a fall (freefall followed by high g forces a second later) and automatically transmit a distress message [6]. Alternatively, a button on the pendant can be pressed. Radio transmissions are used to transmit the distress call from the pendant on the fall alert necklace. These transmissions radiate a relatively large amount of power into free space. This is especially true if cellular signals are used and for long-range transmitters.
A basic problem with the fall detector is that the battery needs to be either replaced at regular intervals, or the device needs to be charged, which can be challenging depending on health circumstances. An alternative approach is to replace the battery with an energy harvester paired with a supercapacitor.
5.2 Solution & Design
In this project, energy harvesting technology is paired with a flexible supercapacitor installed inside a necklace worn by the user to replace the battery inside. Self-powered and battery free, users would not question if their device was ready for use as designed in Figure 5.
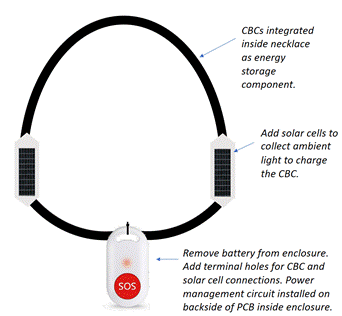
The basic components of this design use small solar panels, CBCs, and a Power Management Integrated Circuit (PMIC) to provide power to the SOS pendant. A typical design uses four of PowerFilm’s ONP2.4-15x94 cells connected in parallel. Each cell provides a maximum of 2.4 volts at 18.6mA (~45mW) in direct sun. These thin solar cells are installed on the necklace above the pendent. The CBCs are installed inside of a neoprene like sleeve. They are flexible enough to curve their way around the neck and are light enough in weight to sit comfortably and not feel cumbersome to the user.
A specialty IC (part #AEM10941) from e-peas harvests energy from the solar panels controlled with a maximum power point tracker (MPPT) and charges the three series connected, 3 Farad CBCs. This PMIC can handle multiple supercapacitors in series and/or parallel. The block diagram for this PMIC can be seen in Figure 6. Our selected solar cells max out at 180 mW under ideal conditions.
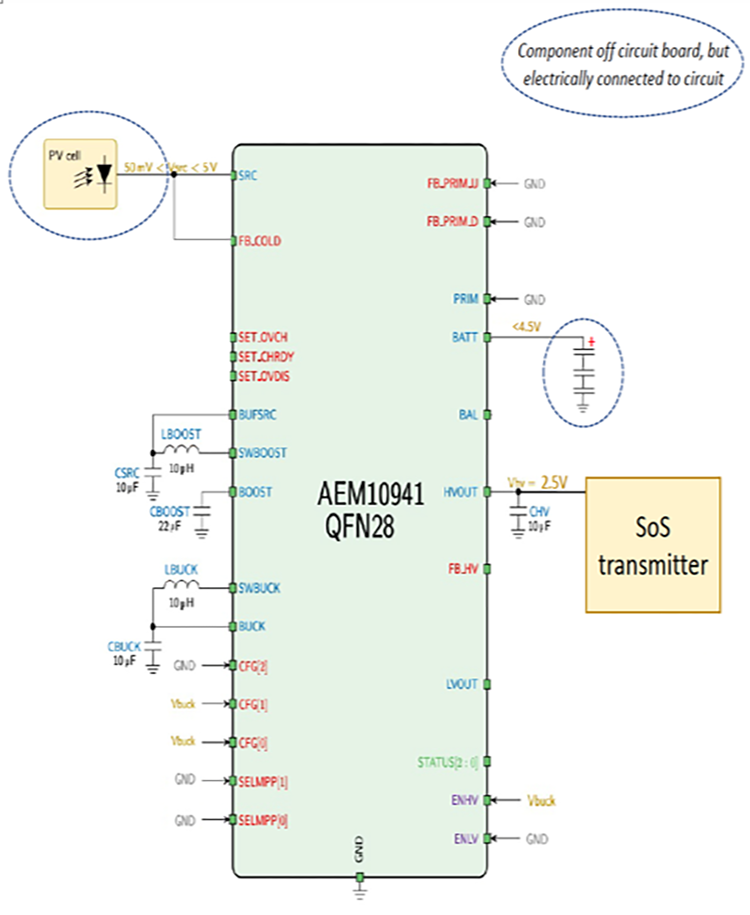
The PMIC also has a Low Drop-Out (LDO) regulator that can put out up to 80mA and can be adjusted to limit output to the 2.5 volts needed by the SOS transmitter. This PMIC also has various configuration options and has been found to work best under the Dual-cell Supercapacitor configuration option.
5.3 Function & Performance
Bright light charges the CBCs quickly to power the device. However, if the indoor light is too dim for an extended period, the voltage can drop below the requirement for the SOS pendent. The CBCs charge even at low light levels of 250 LUX. A well-lit room usually is at about 500 LUX. Direct sunlight is over 10,000 LUX. Even on an overcast day, the sky provides over 1000 LUX and will provide adequate charge for the pendant to operate [7].
These described charging periods can and will include short spells in the sun such as a brisk walk to collect the morning paper or daily mail, a stroll through a local park, driving to the grocery store, working in the garden, or even just relaxing partially in the shade on the porch. Even if direct light is not making contact with the surface of the solar cell, the indirect light from the sun is intense enough to give acceptable light levels for charging. Figure 7, shown below, is a prime example of testing these scenarios for accuracy.
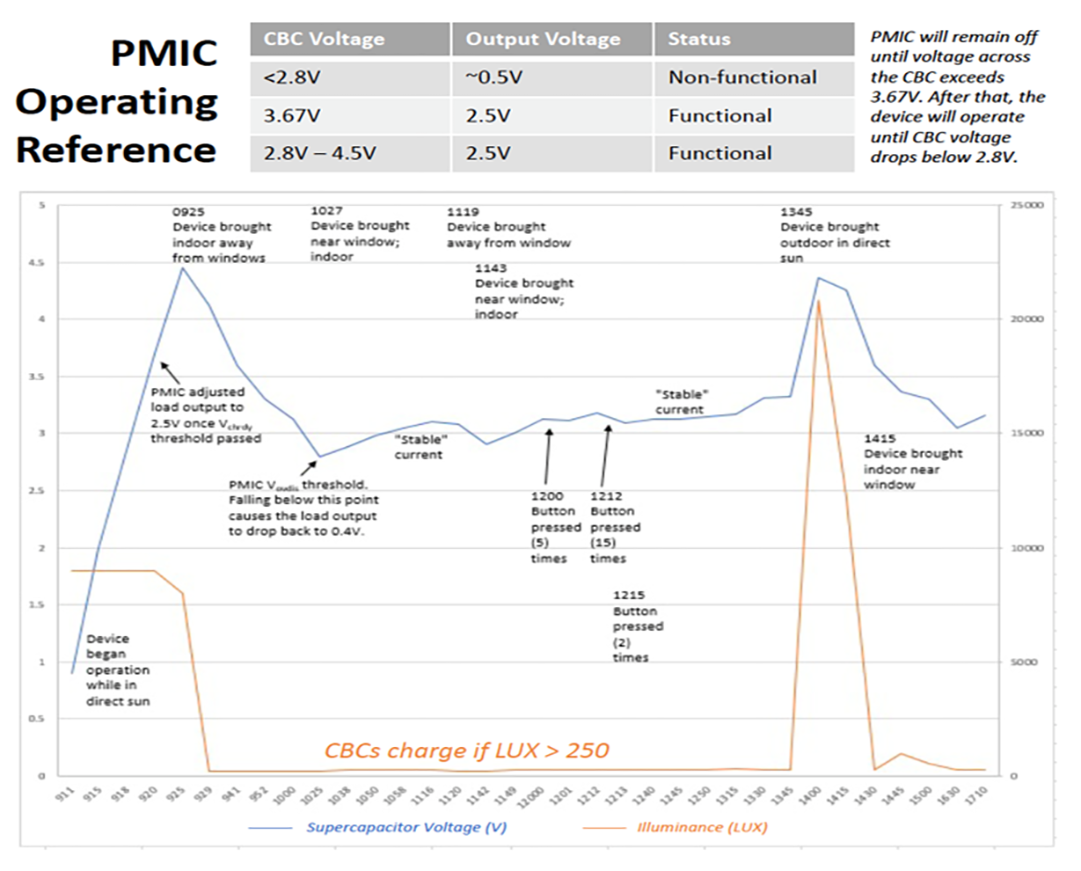
Our results show that the CBC paired with indoor solar cells can replace a coin cell battery enabling battery-free operation of this wearable IoT device. A very small battery can be easily included to serve as a fail-safe backup if used in the dark for an extensive period. Even with a backup battery integrated, the proposed design would still significantly outperform a design using only a battery in terms of service life. While such a design would typically require supercapacitors to be installed on the circuit inside the pendant, which would significantly increase the size of the product worn by users, the CBCs are hidden inside the necklace of the product’s infrastructure to maintain design aesthetics.
5. Conclusion
The Cable-Based Capacitor (CBC) represents a new kind of innovation in the energy storage industry. By focusing on form factor, the CBC is a supercapacitor that offers design engineers new options to improve their products by adding features, optimizing space for miniaturization, complementing and/or replacing batteries, and improving performance.
There are a variety of use cases for the CBC stemming from peak power complement, backup power, and energy harvesting applications. Leveraging the CBC’s form factor, supercapacitors can be installed inside wiring and cabling infrastructure rather than on circuit boards, allowing it to be used in ways previously impossible. Cable-based peak power complement and backup power supplies can be highly useful in exoskeleton suits, automotive auxiliary power supplies, and renewable power systems.
References
[1] Z. Yu and J. Thomas, “Coaxial supercapacitor-electrical cables: Integrating energy storage device into electrical cables,” Adv. Mater. 26, 4279 (2014). View Article
[2] None. (2020, December 08). BU-209: How does a Supercapacitor Work? [Online]. Available: View Article
[3] Powerfilm Solar, “Electronic Component Solar Panels: Indoor Light Series”, datasheet, [Online]. Available: View Article
[4] Keysight Technologies, “Battery Life is IOT Device Life.”, Sept. 25, 2018 [Online]. Available: View Article
[5] H. Akinaga, “Recent Advances and Future Prospects in Energy Harvesting Technologies.” Japanese Journal of Applied Physics, vol. 59, no. 11, Nov. 2020 View Article
[6] S. Jung, S. Hong, J. Kim, S. Lee, T. Hyeon, M. Lee, D. Kim, “Wearable Fall Detector using Integrated Sensors and Energy Devices.” Scientific Reports 5, 17081 (2015). View Article
[7] S. Biswas and H. Kim, “Solar Cells for Indoor Applications: Progress and Development.” Polymers vol. 12,6 1338. 12 Jun. 2020, View Article